# Magnetic Materials: Your Comprehensive Guide to Properties, Types & Applications
This guide provides a deep dive into the fascinating world of magnetic materials, exploring their fundamental properties, diverse classifications, and widespread applications across various industries. Whether you’re a student, engineer, or simply curious about how magnetism shapes our technology, this article will equip you with a solid understanding of these essential materials.
## Fundamental Properties of Magnetic Materials
Magnetic materials are characterized by their ability to create magnetic fields or respond to external magnetic fields. This behavior arises from the intrinsic magnetic moments of their constituent atoms, primarily due to the spin of electrons. Key properties that define magnetic materials include permeability, coercivity, saturation magnetization, and Curie temperature.
Permeability describes a material’s ability to conduct magnetic flux. A high permeability indicates that the material can easily be magnetized and concentrate magnetic field lines. Coercivity, on the other hand, measures the resistance of a magnetic material to demagnetization. A high coercivity signifies that the material retains its magnetism even in the presence of opposing magnetic fields. Saturation magnetization refers to the maximum magnetic moment that can be induced in a material when subjected to a strong external magnetic field. This value represents the point where all the magnetic domains within the material are aligned in the same direction.
Finally, the Curie temperature (Tc) is the critical temperature above which a ferromagnetic or ferrimagnetic material loses its spontaneous magnetization and transitions to a paramagnetic state. At temperatures below Tc, the atomic magnetic moments are aligned, leading to a strong macroscopic magnetic moment. Above Tc, thermal energy overcomes the aligning forces, causing the moments to become randomly oriented and the material to lose its ferromagnetic properties.
## Diamagnetism, Paramagnetism, and Ferromagnetism: An Overview
Magnetic materials can be broadly classified into three main categories based on their interaction with external magnetic fields: diamagnetic, paramagnetic, and ferromagnetic. Each class exhibits distinct magnetic behavior due to the arrangement and interaction of their atomic magnetic moments.
Diamagnetic materials are weakly repelled by magnetic fields. They have no permanent magnetic moments in the absence of an external field. When exposed to a magnetic field, the electron orbits within the atoms are slightly altered, inducing a small magnetic moment that opposes the applied field. Examples of diamagnetic materials include copper, gold, and water.
Paramagnetic materials are weakly attracted by magnetic fields. Unlike diamagnetic materials, they possess permanent atomic magnetic moments. However, these moments are randomly oriented in the absence of a magnetic field, resulting in no net magnetization. When exposed to a magnetic field, the atomic moments tend to align with the field, producing a weak magnetization in the same direction. Examples of paramagnetic materials include aluminum, titanium, and oxygen.
Ferromagnetic materials exhibit strong magnetism and are strongly attracted by magnetic fields. They possess permanent atomic magnetic moments that are aligned even in the absence of an external field, resulting in spontaneous magnetization. This alignment occurs due to strong exchange interactions between the atomic moments. Below the Curie temperature, ferromagnetic materials maintain their magnetization. Examples of ferromagnetic materials include iron, nickel, and cobalt. These materials are widely used in transformers, motors, and magnetic storage devices.
## Understanding Antiferromagnetism and Ferrimagnetism
Beyond the three primary categories (diamagnetism, paramagnetism, and ferromagnetism), there are two other important classes of magnetic materials: antiferromagnetic and ferrimagnetic materials. These materials exhibit unique magnetic ordering due to the presence of multiple sublattices with opposing magnetic moments.
Antiferromagnetic materials possess ordered atomic magnetic moments that are aligned in an antiparallel fashion. While the individual moments are strong, the opposing alignment results in zero net magnetization. Below a specific temperature called the Néel temperature (TN), the antiparallel ordering is stable. Above TN, thermal energy disrupts the ordering, and the material becomes paramagnetic. Antiferromagnetic materials are not commonly used in direct applications, but they form the basis for more complex magnetic materials like ferrimagnets.
Ferrimagnetic materials are similar to antiferromagnetic materials in that they also possess multiple sublattices with opposing magnetic moments. However, the magnetic moments in each sublattice are not equal. This inequality results in a net spontaneous magnetization, similar to ferromagnetic materials, but with a weaker overall magnetic moment. Ferrimagnetic materials are commonly used in high-frequency applications, such as inductors and transformers, due to their high resistivity and low eddy current losses. A common example is ferrite (iron oxide).
## Hard vs Soft Magnetic Materials: Differentiating Properties
Magnetic materials can be further categorized as either hard or soft, based on their coercivity and other magnetic properties. This classification is critical for selecting the appropriate material for specific applications.
Hard magnetic materials, also known as permanent magnets, have high coercivity and high remanence (the magnetization remaining after the external field is removed). These materials are difficult to demagnetize, making them ideal for applications requiring a stable magnetic field, such as electric motors, generators, and magnetic data storage. Common examples include Alnico magnets (aluminum, nickel, cobalt), ferrite magnets, and rare-earth magnets like neodymium magnets (NdFeB) and samarium-cobalt magnets (SmCo). The high coercivity of hard magnets makes them resistant to external demagnetizing fields and temperature variations.
Soft magnetic materials, on the other hand, have low coercivity and high permeability. These materials are easily magnetized and demagnetized, making them suitable for applications where the magnetic field needs to be rapidly changed or switched, such as transformer cores, inductors, and magnetic shielding. Common examples include iron, silicon steel, Permalloy (nickel-iron alloy), and amorphous alloys. The low coercivity of soft magnets allows them to respond quickly to changes in the applied magnetic field, minimizing energy losses due to hysteresis.
## Applications in Data Storage: From Tapes to Hard Drives
Magnetic materials are fundamental to data storage technologies, enabling the recording and retrieval of information in various forms, from magnetic tapes to hard disk drives (HDDs) and magnetic random-access memory (MRAM).
Magnetic tapes utilize a thin coating of magnetic material, such as iron oxide or chromium dioxide, deposited on a plastic film. Data is recorded by aligning the magnetic domains in the coating along the length of the tape. Magnetic tape is still used for archival storage due to its high capacity and relatively low cost.
Hard disk drives (HDDs) employ rotating platters coated with a thin layer of magnetic material. Data is written and read by a magnetic head that flies above the platter surface. The magnetic domains on the platter are aligned to represent binary data (0s and 1s). The density of data storage increases as the size of the magnetic domains decreases, requiring materials with higher coercivity and smaller grain size.
Magnetic Random-Access Memory (MRAM) is a non-volatile memory technology that uses magnetic storage elements to store data. In MRAM, data is stored as the magnetic orientation of two ferromagnetic layers separated by a thin insulating layer. The resistance across the layers depends on the relative orientation of their magnetic moments, a phenomenon known as tunneling magnetoresistance (TMR). MRAM offers several advantages over conventional RAM including non-volatility, high speed, and low power consumption.
## Magnetic Materials in Motors and Generators
Electric motors and generators rely heavily on magnetic materials to convert electrical energy into mechanical energy and vice versa. These devices utilize the interaction between magnetic fields and electric currents to produce motion or generate electricity.
In electric motors, magnetic fields are generated by permanent magnets or electromagnets (coils of wire wound around a ferromagnetic core). The interaction between these magnetic fields and the current-carrying conductors in the motor creates a force that causes the rotor to rotate. Hard magnetic materials with high coercivity and high remanence are preferred for permanent magnet motors, as they provide a strong and stable magnetic field without the need for continuous electrical input. Soft magnetic materials are used in the stator core to enhance the magnetic field strength and reduce energy losses due to hysteresis.
In electric generators, a conductor is moved through a magnetic field, inducing a voltage and causing current to flow. The strength of the generated voltage depends on the strength of the magnetic field and the speed of the conductor’s movement. Similar to motors, generators use both hard and soft magnetic materials. Permanent magnets are used in some generators, while electromagnets with soft magnetic cores are used in larger generators to provide a strong and controllable magnetic field.
## Applications of Magnetic Materials in Medical Technology
Magnetic materials play a crucial role in various medical technologies, including magnetic resonance imaging (MRI), targeted drug delivery, and hyperthermia cancer treatment. These applications leverage the unique magnetic properties of specific materials to diagnose and treat diseases.
Magnetic Resonance Imaging (MRI) is a powerful medical imaging technique that uses strong magnetic fields and radio waves to create detailed images of the organs and tissues in the body. The patient is placed inside a strong magnetic field, which aligns the magnetic moments of the protons in the body’s water molecules. Radio waves are then used to excite these protons, and the signals emitted by the protons are detected and processed to create an image. Superconducting magnets made from materials like niobium-titanium alloy are used to generate the strong magnetic fields required for MRI.
Targeted drug delivery utilizes magnetic nanoparticles coated with drugs to deliver medication directly to the site of a disease. The nanoparticles are injected into the body and guided to the target area using an external magnetic field. This technique minimizes the side effects of drugs by reducing their exposure to healthy tissues. Magnetite (Fe3O4) nanoparticles are commonly used for targeted drug delivery due to their biocompatibility and ease of magnetic manipulation.
Hyperthermia cancer treatment involves heating cancerous tissues to temperatures above 41°C to kill cancer cells. Magnetic nanoparticles are injected into the tumor and then heated by applying an alternating magnetic field. The heat generated by the nanoparticles selectively destroys the cancer cells while sparing the surrounding healthy tissues. Iron oxide nanoparticles are frequently used in hyperthermia cancer treatment due to their high heating efficiency and biological safety.
## Emerging Trends and Future Directions in Magnetic Materials
The field of magnetic materials is constantly evolving, with researchers exploring new materials, nanostructures, and applications. Several emerging trends and future directions promise to revolutionize various industries.
One key trend is the development of advanced magnetic nanocomposites. These materials combine magnetic nanoparticles with other materials, such as polymers or ceramics, to create new functionalities and tailored properties. Magnetic nanocomposites are being explored for applications in sensors, actuators, and energy storage.
Another important area of research is the development of spintronic devices. Spintronics, also known as spin electronics, utilizes the spin of electrons to store and process information. Spintronic devices offer the potential for faster, smaller, and more energy-efficient electronics compared to conventional charge-based electronics.
Research is also focused on developing new and improved permanent magnets that are less reliant on rare-earth elements. Rare-earth elements are critical components of high-performance permanent magnets, but their supply is limited and geographically concentrated. Researchers are exploring alternative materials and processing techniques to create magnets with comparable performance using more abundant elements.
**Заключение**
Magnetic materials are integral to countless technological applications, spanning data storage, energy generation, medical technology, and beyond. Understanding their properties, types, and applications is crucial for engineers, scientists, and anyone seeking to grasp the inner workings of modern technology. From the fundamental principles of diamagnetism, paramagnetism, and ferromagnetism to the complexities of antiferromagnetism and ferrimagnetism, and from the practical uses of hard and soft magnets to the cutting-edge research in magnetic nanocomposites and spintronics, this guide provides a comprehensive overview of this fascinating field. The future of magnetic materials promises even greater advancements, shaping the technologies of tomorrow.
## FAQ
### What are the key differences between hard and soft magnetic materials?
Hard magnetic materials have high coercivity and high remanence, making them difficult to demagnetize. They are used for permanent magnets. Soft magnetic materials have low coercivity and high permeability, making them easy to magnetize and demagnetize. They are used in transformers and inductors.
### What is the Curie temperature, and why is it important?
The Curie temperature (Tc) is the temperature above which a ferromagnetic or ferrimagnetic material loses its spontaneous magnetization and transitions to a paramagnetic state. It’s important because it sets the upper limit for the operating temperature of magnetic devices.
### What are some common applications of magnetic materials in the medical field?
Magnetic materials are used in MRI for imaging, targeted drug delivery to deliver medication directly to diseased areas, and hyperthermia cancer treatment to heat and destroy cancer cells.
### What are some emerging trends in magnetic materials research?
Emerging trends include the development of magnetic nanocomposites, spintronic devices, and new permanent magnets that are less reliant on rare-earth elements.
### How does the choice of magnetic material affect the performance of an electric motor?
The choice of magnetic material significantly impacts the performance of an electric motor. Hard magnetic materials with high coercivity and high remanence are used for permanent magnet motors, providing a strong and stable magnetic field. Soft magnetic materials are used in the stator core to enhance the magnetic field strength and reduce energy losses.
### What role do magnetic materials play in data storage?
Magnetic materials are fundamental to data storage, enabling the recording and retrieval of information in various forms. They are used in magnetic tapes, hard disk drives (HDDs), and magnetic random-access memory (MRAM). The magnetic orientation of domains represents binary data (0s and 1s).
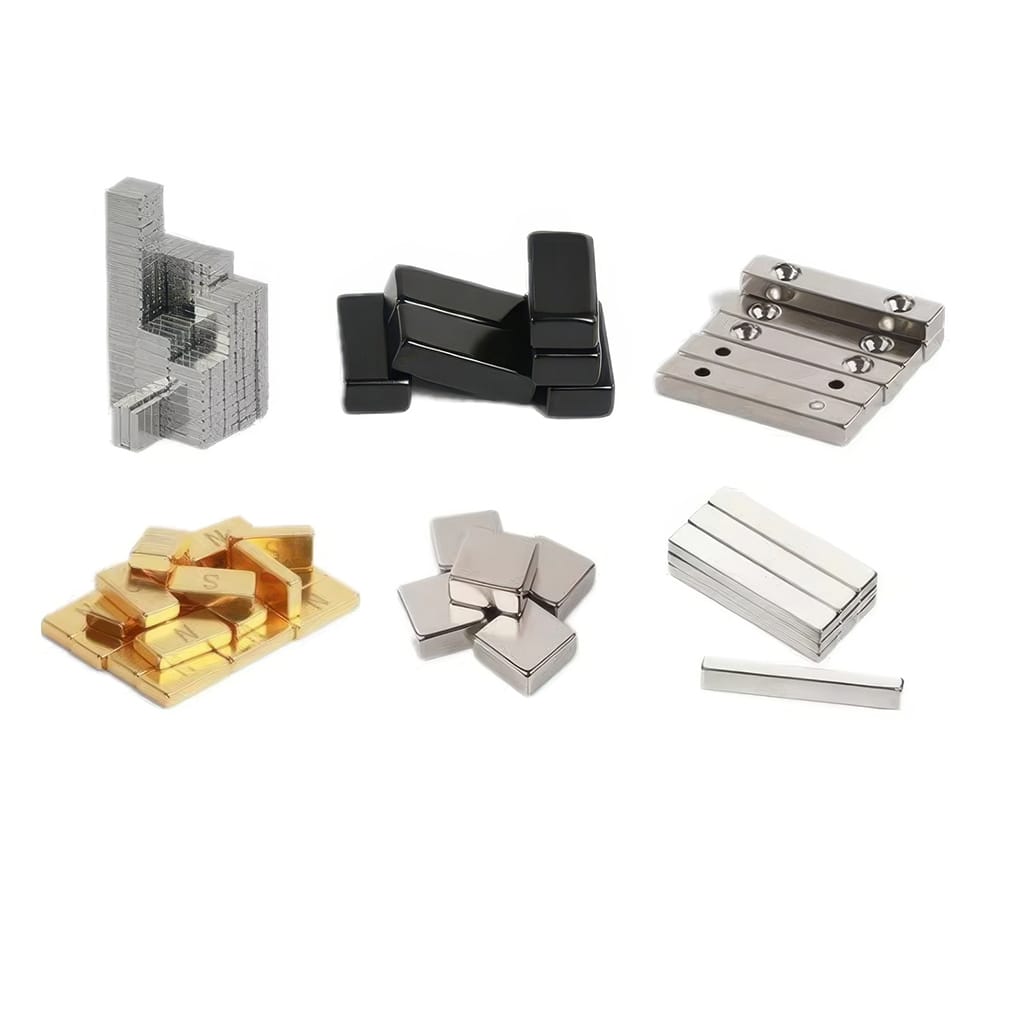