# Top Magnetic Materials: A Comprehensive Guide for Engineers and Enthusiasts
**Summary:** This article provides a detailed overview of the most important magnetic materials used in various engineering and scientific applications. From fundamental concepts to specific material properties, applications, and future trends, this guide offers valuable insights for engineers, hobbyists, and anyone interested in magnetism. Learn about the characteristics, advantages, and limitations of each material to make informed decisions for your projects.
## Ferromagnetic Materials: The Foundation of Magnetism
Ferromagnetic materials are the most widely recognized type of magnetic material. They exhibit strong magnetism in the presence of an external magnetic field and retain a significant amount of magnetism even after the field is removed. This characteristic, known as hysteresis, makes them ideal for permanent magnets and applications requiring strong magnetic fields. The alignment of magnetic domains within the material is responsible for this behavior.
Iron, nickel, and cobalt, along with their alloys, are the most common examples of ferromagnetic materials. Their atomic structure allows for strong magnetic moments to align collectively, creating a powerful macroscopic magnetic field. Different alloy compositions can tailor the magnetic properties, such as coercivity (resistance to demagnetization) and remanence (residual magnetization), for specific applications.
Beyond these core elements, alloys like Alnico (Aluminum, Nickel, Cobalt, and Iron) and various steel alloys showcase the versatility of ferromagnetic materials. Alnico, known for its high coercivity and good temperature stability, is used in motors, generators, and sensors. Steel alloys, with their varying carbon content and added elements, offer a range of properties, from soft magnetic steels used in transformer cores to hard magnetic steels used in permanent magnets.
## Ferrimagnetic Materials: A Balance of Magnetism
Ferrimagnetic materials, while seemingly similar to ferromagnetic materials, possess a crucial difference in their atomic structure. Unlike ferromagnetic materials where all magnetic moments align in the same direction, in ferrimagnetic materials, magnetic moments within different atomic sites align in *opposite* directions. However, these opposing moments are not equal, resulting in a net magnetic moment and thus, exhibiting strong magnetism.
Ferrites, a class of ceramic materials composed of iron oxide and other metallic elements like manganese, zinc, or nickel, are the most prominent examples of ferrimagnetic materials. Importantly, they are insulators, making them ideal for high-frequency applications where eddy current losses are a concern. This is a significant advantage over ferromagnetic metals, which are excellent conductors and suffer from significant energy losses at high frequencies.
The specific composition of the ferrite determines its magnetic properties. For instance, manganese-zinc ferrites are often used in inductors and transformers operating at lower frequencies, while nickel-zinc ferrites are favored for higher-frequency applications. The development of specialized ferrites has been crucial for advancements in telecommunications, electronics, and power electronics.
## Paramagnetic Materials: Weakly Magnetic Responders
Paramagnetic materials are characterized by their weak attraction to magnetic fields. Unlike ferromagnetic materials, they do not retain any magnetism when the external field is removed. This behavior arises from the presence of unpaired electrons within the material’s atoms. These unpaired electrons possess magnetic moments that align randomly in the absence of an external field, resulting in no net magnetization.
When an external magnetic field is applied, the magnetic moments of the unpaired electrons tend to align with the field, creating a small, temporary magnetization. This magnetization is proportional to the applied field strength and the magnetic susceptibility of the material. The higher the magnetic susceptibility, the stronger the induced magnetization.
Examples of paramagnetic materials include aluminum, platinum, oxygen, and magnesium. Though their individual magnetic responses are weak, these materials are crucial in certain applications. For example, oxygen’s paramagnetism is utilized in medical oxygen sensors. Furthermore, paramagnetic materials can be doped into other materials to modify their magnetic properties.
## Diamagnetic Materials: Repelled by Magnetic Fields
Diamagnetic materials exhibit a unique property: they are repelled by magnetic fields. This phenomenon arises from the fact that the application of an external magnetic field induces circulating electric currents within the atoms of the material. These circulating currents create a magnetic field that opposes the applied field, resulting in a repulsive force.
Unlike paramagnetic materials, diamagnetic materials do not possess unpaired electrons. The magnetic moments are induced by the external field, rather than being inherent to the material. The strength of the repulsion is relatively weak and is proportional to the strength of the applied field.
Examples of diamagnetic materials include copper, gold, silver, bismuth, and water. Although their repulsive force is weak, diamagnetism plays a role in various applications. Magnetic levitation, for instance, can be achieved using diamagnetic materials in conjunction with strong magnetic fields. Diamagnetic shielding is also used in sensitive electronic equipment to protect them from external magnetic interference.
## Soft Magnetic Materials: Easily Magnetized and Demagnetized
Soft magnetic materials are distinguished by their ability to be easily magnetized and demagnetized. They possess low coercivity and low remanence, meaning they require only a small external field to become magnetized, and they quickly lose their magnetism when the field is removed. This characteristic makes them ideal for applications where magnetic fields need to be switched rapidly, such as in transformers, inductors, and electromagnets.
The microstructure of soft magnetic materials plays a crucial role in their magnetic behavior. Generally, they have a fine-grained structure with minimal internal stresses. This allows for easy movement of magnetic domain walls, which are the boundaries between regions of uniform magnetization within the material. The easier the domain walls can move, the lower the energy required to magnetize and demagnetize the material.
Common examples of soft magnetic materials include silicon steel, nickel-iron alloys (such as Permalloy and Mu-metal), and soft ferrites. Silicon steel, used extensively in transformer cores, exhibits excellent permeability and low hysteresis losses. Nickel-iron alloys possess extremely high permeability and are used in sensitive magnetic shielding applications. Soft ferrites, being insulators, minimize eddy current losses at high frequencies.
## Hard Magnetic Materials: Maintaining Strong Magnetization
Hard magnetic materials, also known as permanent magnets, are characterized by their high coercivity and high remanence. They are difficult to magnetize and demagnetize, allowing them to retain a strong magnetic field even in the presence of opposing fields or demagnetizing influences. This makes them suitable for applications requiring a constant magnetic field, such as in electric motors, generators, magnetic bearings, and various holding applications.
The high coercivity of hard magnetic materials is attributed to their microstructure, which hinders the movement of magnetic domain walls. These materials often contain defects, grain boundaries, or precipitates that act as pinning sites, making it difficult for the domain walls to move in response to an external field. The larger the energy required to move the domain walls, the greater the coercivity.
Examples of hard magnetic materials include Alnico, ferrite magnets (hard ferrites), rare-earth magnets (such as neodymium magnets and samarium-cobalt magnets), and certain hardened steel alloys. Rare-earth magnets boast the highest energy product (a measure of the magnet’s strength) and are used in high-performance motors and generators. Alnico, with its good temperature stability, finds use in sensors and older motor designs. Hard ferrites are cost-effective and prevalent in loudspeakers and various industrial applications.
## Rare-Earth Magnets: Powerhouses of Magnetism
Rare-earth magnets represent the pinnacle of magnetic performance, achieving the highest energy product among all permanent magnet materials. They are based on alloys of rare-earth elements, primarily neodymium (Nd) and samarium (Sm), with iron (Fe) and boron (B) for neodymium magnets (NdFeB) and cobalt (Co) for samarium-cobalt magnets (SmCo). These magnets offer exceptional strength and are essential for numerous modern technologies.
Neodymium magnets, or NdFeB magnets, are the most widely used type of rare-earth magnet. They offer the highest energy product for their size and cost, making them ideal for applications requiring high performance in a compact form. They are commonly found in electric vehicle motors, hard disk drives, wind turbine generators, and various consumer electronics. However, neodymium magnets are susceptible to corrosion and can lose their magnetism at elevated temperatures.
Samarium-cobalt magnets, or SmCo magnets, offer superior temperature stability and corrosion resistance compared to neodymium magnets. While they have a slightly lower energy product than NdFeB magnets, their robustness at high temperatures makes them suitable for applications where the magnet is exposed to harsh environmental conditions. They find applications in aerospace, military, and high-temperature motor designs.
## Nanomagnetic Materials: Magnetism on a Microscopic Scale
Nanomagnetic materials represent a frontier in magnetic material research and development. These materials, with at least one dimension in the nanometer scale (1-100 nanometers), exhibit unique magnetic properties that differ significantly from their bulk counterparts. The confinement of magnetic moments within nanoscale dimensions leads to novel phenomena such as superparamagnetism and quantum tunneling of magnetization.
Superparamagnetism arises when the size of the magnetic particles is so small that their magnetization can fluctuate randomly due to thermal energy. This results in zero net magnetization in the absence of an external field, but a strong response to an applied field. Superparamagnetic nanoparticles are used in magnetic resonance imaging (MRI), targeted drug delivery, and magnetic data storage.
Quantum tunneling of magnetization refers to the phenomenon where the magnetization of a nanomagnet can spontaneously flip direction through a potential energy barrier. This effect is particularly relevant at very low temperatures and can impact the stability of magnetic data storage devices. The study of nanomagnetic materials is paving the way for new technologies with enhanced capabilities.
## Amorphous Magnetic Materials: Disordered Structures with Unique Properties
Amorphous magnetic materials, also known as metallic glasses, are characterized by their disordered atomic structure. Unlike crystalline magnetic materials with their well-defined crystal lattices, amorphous materials lack long-range order. This unique structure leads to several advantageous properties, including high permeability, low coercivity, and low core losses.
The absence of grain boundaries in amorphous magnetic materials minimizes the energy required for domain wall movement, resulting in lower hysteresis losses. Furthermore, their high resistivity reduces eddy current losses, making them suitable for high-frequency applications. These properties make them attractive for transformer cores, magnetic shielding, and sensors.
Amorphous magnetic materials are typically produced using rapid solidification techniques, such as melt-spinning, which prevent the atoms from arranging into a crystalline structure. Common examples include alloys based on iron, cobalt, and nickel with metalloid elements like boron, silicon, and phosphorus. Their unique combination of properties makes them suitable for specialized applications where low losses and high permeability are critical.
## Conclusion
This comprehensive guide has explored a range of magnetic materials, from the foundational ferromagnetic substances to the advanced nanomagnetic structures. Each material offers a unique set of properties, making it suitable for specific applications across various engineering disciplines. Understanding the characteristics, advantages, and limitations of these materials is crucial for engineers and enthusiasts alike to design innovative and efficient devices and systems. As technology continues to advance, research and development in magnetic materials will undoubtedly lead to even more sophisticated and groundbreaking applications in the future. This detailed exploration helps engineers make informed choices when selecting materials for their projects, optimizing performance and functionality. Whether building small electronics, designing high-power systems, or exploring novel magnetic phenomena, the information outlined in this guide serves as a valuable reference point for navigating the world of magnetic materials.
## FAQ
### ### H3: What is magnetic susceptibility?
Magnetic susceptibility is a measure of how easily a material becomes magnetized in response to an applied magnetic field. A positive susceptibility indicates a paramagnetic material, a negative susceptibility indicates a diamagnetic material, and a very large positive susceptibility indicates a ferromagnetic or ferrimagnetic material.
### ### H3: What is coercivity?
Coercivity is a measure of a magnetic material’s resistance to demagnetization. It is the strength of the magnetic field required to reduce the magnetization of a material to zero after it has been saturated. Hard magnetic materials have high coercivity, while soft magnetic materials have low coercivity.
### ### H3: What is remanence?
Remanence is the magnetization that remains in a magnetic material after the external magnetic field has been removed. Hard magnetic materials have high remanence, retaining a strong magnetic field even without an external field, while soft magnetic materials have low remanence.
### ### H3: What is the difference between ferromagnetic and ferrimagnetic materials?
While both ferromagnetic and ferrimagnetic materials exhibit strong magnetism, their atomic structures differ. In ferromagnetic materials, all magnetic moments align in the same direction, resulting in a large net magnetization. In ferrimagnetic materials, magnetic moments align in opposite directions on different atomic sites, but the moments are unequal, resulting in a net magnetization.
### ### H3: What are the advantages of using ferrites compared to ferromagnetic metals at high frequencies?
Ferrites are insulators, while ferromagnetic metals are conductors. At high frequencies, conductive materials experience eddy current losses, which dissipate energy as heat. Ferrites, being insulators, minimize eddy current losses, making them more efficient for high-frequency applications.
### ### H3: What are the limitations of neodymium magnets?
Neodymium magnets, while the strongest permanent magnets available, are susceptible to corrosion and can lose their magnetism at elevated temperatures. Protecting them from moisture and limiting their exposure to high temperatures is crucial for preserving their performance.
### ### H3: What are soft magnetic materials used for?
Soft magnetic materials are used in applications where magnetic fields need to be switched rapidly and efficiently, such as in transformer cores, inductors, and electromagnets. Their low coercivity and low remanence allow them to be easily magnetized and demagnetized.
### ### H3: What factors affect the choice of magnetic material for an application?
Several factors influence the choice of magnetic material for a specific application, including the required magnetic strength, operating temperature, frequency, size constraints, cost, and environmental conditions. Understanding the interplay between these factors is crucial for selecting the optimal material.
### ### H3: What are some emerging trends in magnetic materials research?
Emerging trends in magnetic materials research include the development of new rare-earth-free permanent magnets, the exploration of nanomagnetic materials for advanced data storage and biomedical applications, and the investigation of magnetoelectric materials that exhibit coupling between magnetic and electric properties. These advancements promise to unlock new possibilities in various technological fields.
### ### H3: Where can I learn more about specific magnetic materials and their suppliers?
Reliable sources for further information include academic journals specializing in materials science and engineering, manufacturer datasheets, and technical resources provided by magnetic materials suppliers like Arnold Magnetic Technologies, TDK, and VACUUMSCHMELZE. Consulting with experienced materials engineers can also be beneficial.
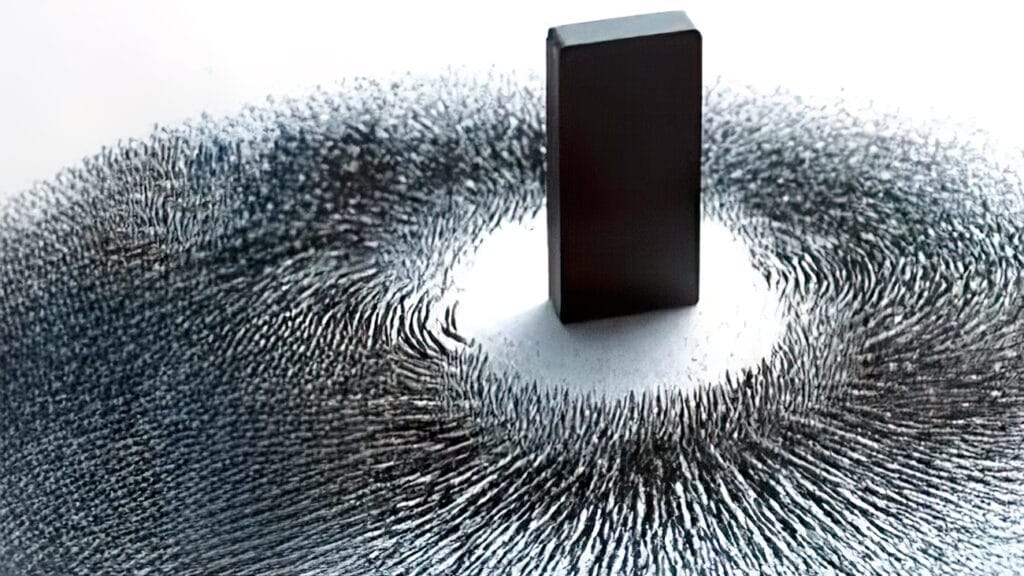