# Scientists Create Magnet So Powerful It Could (Theoretically) Float a Car! Revolutionary Advancement in Magnetic Field Technology
This article dives into the groundbreaking achievement of scientists who have developed a magnet with unprecedented strength. While floating a whole car might be a slight exaggeration for now, the implications of this technology are enormous, impacting fields ranging from medicine and transportation to energy production and fundamental physics research. Read on to understand the science behind this powerful magnet, its potential applications, and the challenges that lie ahead in realizing its full potential.
## The Dawning of Ultra-High Field Magnetism
The pursuit of stronger magnetic fields has been a constant endeavor in scientific research. Stronger magnets allow for more detailed investigations into the fundamental properties of matter, enabling breakthroughs in various fields. Historically, the limitations of magnet strength have been significant roadblocks. This new magnet represents a substantial leap forward, pushing the boundaries of what’s currently possible and opening doors to previously inaccessible experiments and technologies. It’s not just about building a bigger magnet; it’s about fundamentally changing what we can *do* with magnetism.
The creation of this ultra-high field magnet is a testament to advancements in materials science, engineering, and cryogenic technologies. Overcoming the immense stresses and heat generated by such a powerful magnetic field required innovative design and the development of new materials capable of withstanding extreme conditions. This achievement isn’t just about the magnet itself, but also about the synergistic breakthroughs in supporting technologies that made its creation possible.
## Understanding Magnetic Field Strength: Teslas and Beyond
Magnetic field strength is typically measured in Teslas (T). To put this development into perspective, a refrigerator magnet typically produces a magnetic field of around 0.001 T, while a strong MRI machine operates at around 3 T. The most powerful superconducting magnets currently in use for research reach around 45 T. This new magnet significantly surpasses that mark, achieving a field strength that dwarfs existing technologies.
The intensity of a magnetic field dictates its potential applications. Higher field strengths allow for greater control over charged particles, enhance the sensitivity of spectroscopic techniques, and enable the exploration of exotic quantum phenomena. Imagine the ability to manipulate atomic structures with unparalleled precision, or to dramatically improve the resolution of medical imaging. These possibilities become increasingly tangible with the advent of ultra-high field magnets.
## The Materials Science Behind the Breakthrough
The key to achieving such a high magnetic field lies in the combination of advanced superconducting materials. Traditional electromagnets rely on copper wires, which have significant resistance, limiting the achievable magnetic field. Superconducting materials, on the other hand, offer virtually zero resistance to electrical current, allowing for much higher currents and therefore stronger magnetic fields. However, even superconductors have their limits, eventually losing their superconductivity under high magnetic fields or high temperatures.
The research team likely employed a combination of different types of superconductors, such as niobium-tin (Nb3Sn) and yttrium barium copper oxide (YBCO), also known as “high-temperature” superconductors. These materials have different strengths and weaknesses, and strategically layering them within the magnet structure can optimize performance and stability. The precise composition and fabrication techniques are often closely guarded secrets, but the underlying principle is to create a structure that can withstand the immense electromagnetic forces while maintaining superconductivity.
## Engineering Challenges: Containing Immense Forces
Building a magnet of this magnitude is not just about the materials; it’s also about the engineering. The forces generated by the magnetic field are enormous, tending to push the magnet apart. These forces increase exponentially with the field strength, making containment a significant challenge. Imagine trying to hold together something that wants to explode with the force of several tons.
The researchers likely employed sophisticated mechanical support structures to counteract these forces. These structures might include high-strength steel casings, pre-stressed composite materials, and innovative winding techniques that distribute the forces evenly across the magnet structure. Advanced computer modeling is crucial for predicting stress distributions and optimizing the design to prevent catastrophic failure. The precision and expertise required for this type of engineering are truly remarkable.
## Cryogenic Cooling: Taming the Heat
Superconductivity only occurs at extremely low temperatures. Therefore, the magnet must be cryogenically cooled using liquid helium or other cryogenic fluids. Maintaining these ultra-low temperatures requires sophisticated cooling systems and insulation to minimize heat leaks. The efficiency and reliability of the cryogenic system are critical for the sustained operation of the magnet.
The cooling process itself can be challenging. Rapid cooling can induce thermal stresses within the magnet structure, potentially damaging the sensitive superconducting materials. Therefore, the cooling process must be carefully controlled to avoid these issues. Furthermore, the cost and availability of cryogenic fluids like liquid helium can be a limiting factor in the widespread deployment of these technologies.
## Potential Applications in Medicine: Enhanced MRI and Beyond
One of the most promising applications of ultra-high field magnets is in medical imaging. Higher magnetic fields in MRI (Magnetic Resonance Imaging) machines can significantly improve image resolution and contrast, allowing doctors to detect smaller tumors and diagnose diseases earlier. This could lead to more effective treatments and improved patient outcomes.
Beyond MRI, ultra-high field magnets could also be used in other medical applications, such as targeted drug delivery. By attaching drugs to magnetic nanoparticles, researchers could use magnetic fields to guide the drugs to specific locations in the body, minimizing side effects and maximizing therapeutic efficacy. This approach holds great promise for treating cancer and other diseases.
## Revolutionizing Energy Production: Fusion and Beyond
Another potential application is in nuclear fusion research. Fusion reactors require extremely strong magnetic fields to confine the hot plasma in which fusion reactions occur. Stronger magnets could lead to smaller, more efficient, and more cost-effective fusion reactors, potentially paving the way for a clean and sustainable energy source.
Furthermore, these magnets could also improve the efficiency of existing energy technologies. For example, they could be used in more efficient electric motors and generators, reducing energy consumption and greenhouse gas emissions. The possibilities for using these advanced magnets in energy-related applications are vast and potentially transformative.
## Fundamental Physics Research: Unveiling the Secrets of the Universe
Beyond practical applications, ultra-high field magnets are invaluable tools for fundamental physics research. They allow scientists to probe the properties of matter under extreme conditions, revealing new insights into the nature of reality. For instance, they can be used to study the behavior of electrons in exotic materials, potentially leading to the discovery of new quantum phenomena.
By creating conditions that mimic the extreme environments found in stars and other astrophysical objects, these magnets can also help scientists understand the fundamental processes that govern the universe. This type of research is essential for advancing our understanding of the world around us and pushing the boundaries of human knowledge.
## Challenges and Future Directions: Scaling Up and Reducing Costs
While this new magnet represents a significant breakthrough, there are still challenges to overcome before it can be widely deployed. One of the main challenges is the cost. The materials and fabrication techniques required to build such a powerful magnet are expensive, limiting its accessibility to only a few research institutions.
Another challenge is the size and weight of the magnet. While the magnetic field strength is impressive, the magnet itself may be too large and heavy for some applications. Future research will focus on miniaturizing the technology and reducing its weight without sacrificing performance. Furthermore, improving the reliability and robustness of the magnet is crucial for ensuring its long-term viability. As technology advances, expect more compact and more powerful magnet technologies, leading to even more transformative applications.
## Conclusion
This newly developed ultra-high field magnet marks a pivotal moment in the advancement of magnetic technology. While the initially eye-catching claim of floating a car might be a future aspiration (or a carefully controlled demonstration), the realistic implications of this magnet’s creation are substantial. The advancements in materials science, engineering, and cryogenic technology that made this magnet possible are revolutionary. With potential applications spanning medicine (enhanced MRI), energy production (fusion reactors), and fundamental physics (exploring quantum realms), this technology promises to reshape numerous fields. Ongoing research and development will focus on reducing costs, improving reliability, and scaling down the size of these magnets, paving the way for broad deployment and unlocking even more transformative possibilities in the years to come.
## FAQ
### What is a Tesla and why is it important?
A Tesla (T) is the standard unit of measurement for magnetic field strength. The higher the Tesla value, the stronger the magnetic field. Stronger magnetic fields allow for more precise manipulation of charged particles, enhanced sensitivity in advanced technologies, and exploration of extreme physical phenomena.
### What are superconducting magnets and why are they used?
Superconducting magnets utilize materials that offer virtually zero resistance to electrical current when cooled to extremely low temperatures. This allows for much higher currents and, consequently, stronger magnetic fields compared to traditional electromagnets made with copper wires. They are essential for achieving high magnetic field strengths.
### What are the main challenges in building ultra-high field magnets?
The primary challenges include containing the immense forces generated by the magnetic field, maintaining cryogenic temperatures to ensure superconductivity, and dealing with the cost and availability of necessary materials and cooling agents. Scaling down the size and weight of the magnet while preserving its performance is also crucial for expanding its applications.
### Is it really possible to float a car with a magnet?
The idea of levitating a car with a magnet is more of a thought experiment to illustrate the magnet’s power. While theoretically possible under ideal circumstances with a sufficiently strong magnetic field and a diamagnetic vehicle, it’s not currently feasible due to the weight of a car and the limitations of current technology. The magnet’s strength is more impactful in facilitating advanced research and technologies.
### What are the potential applications of this new magnet in medicine?
The magnet can significantly improve the resolution and contrast of MRI images, allowing for earlier detection of diseases. It could also be used for targeted drug delivery by guiding magnetic nanoparticles to specific locations in the body.
### How could ultra-high field magnets contribute to energy production?
They can enable more efficient nuclear fusion reactors and enhance the performance of electric motors and generators. Fusion reactors need strong magnetic fields to confine plasma. Stronger magnets allow for more efficient plasma confinement and therefore more viable fusion power.
### What are the implications of this magnet for fundamental physics research?
It enables scientists to study the behavior of matter under extreme conditions and allows for the exploration of exotic quantum phenomena that are not accessible with weaker magnetic fields. These studies contribute to a deeper understanding of the universe and its fundamental laws.
### What is the next step in this research?
Future endeavors will focus on reducing the cost, enhancing the reliability, and minimizing the size of these magnets. Improving efficiency and exploring even stronger superconducting materials are also key areas of research. Making the technology more accessible and practical for a wider range of applications is the ultimate goal.
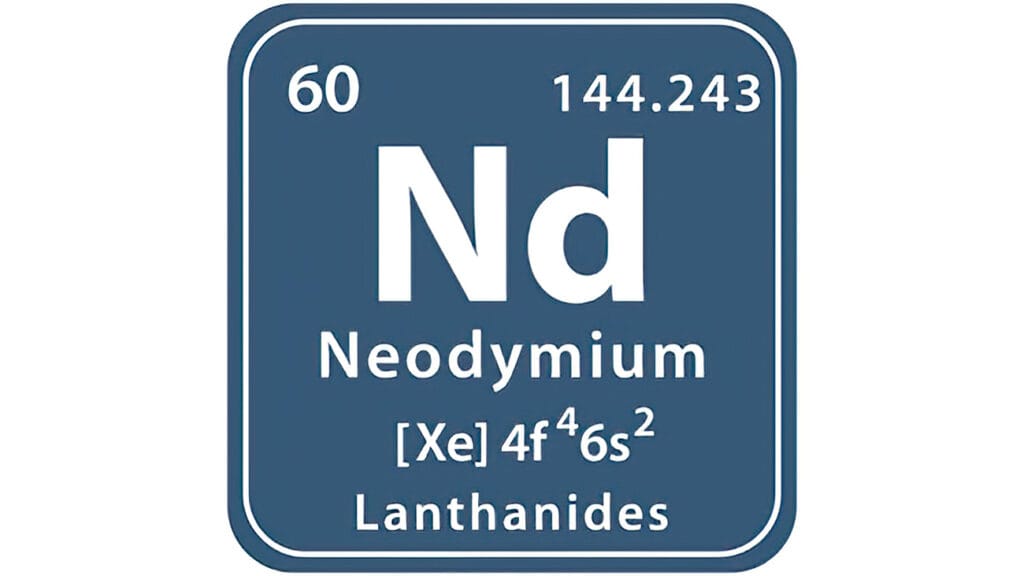