# The Compelling Science of Magnetic Fasteners: Unlocking Their Surprising Strength
**Summary:** This article delves into the fascinating science behind magnetic fasteners, exploring the fundamental principles that govern their incredible strength. We’ll unravel the mysteries of magnetism, examine different types of magnets, and dissect the factors that contribute to a fastener’s holding power. Whether you’re a DIY enthusiast, an engineer, or simply curious about the world around you, this comprehensive guide will equip you with a deeper understanding of how magnetic fasteners work and why they’re so effective. We will explore atomic alignment, magnetic domains, materials science, and design considerations—ultimately explaining what makes these seemingly simple devices so remarkably robust.
## The Atomic Origins of Magnetism: Where It All Begins
Magnetism, at its core, is an atomic phenomenon. It arises from the movement of electrically charged particles – specifically, electrons orbiting atomic nuclei and the intrinsic spin of these electrons. While electrons moving around the nucleus are part of the equation, it’s primarily the electron spin that contributes to a material’s magnetic properties. Each electron behaves as if it’s spinning, generating a tiny magnetic field. In most materials, these tiny magnetic fields are randomly oriented, effectively canceling each other out, resulting in no overall magnetism.
However, in certain materials, particularly ferromagnetic materials like iron, nickel, and cobalt, a unique situation arises. The electron spins spontaneously align themselves in specific regions called magnetic domains. Within each domain, the magnetic fields of the individual atoms reinforce each other, creating a strong collective magnetic field. These domains are still randomly oriented across the entire material, so initially, there is no net magnetic force. However, when exposed to an external magnetic field, these domains tend to align themselves with the external field, leading to the material becoming magnetized.
The strength of the resulting magnetism depends on several factors, including the material’s atomic structure, the extent to which the domains can align with the external field, and the magnitude of the applied field. The ability of these domains to easily align and remain aligned, even after the external field is removed, determines whether a material is a good candidate for permanent magnets used in magnetic fasteners.
## Magnetic Domains: The Microscopic Powerhouses
As mentioned, magnetic domains are regions within a ferromagnetic material where the magnetic moments of atoms are aligned. These domains range in size from a few micrometers to a few millimeters and act as miniature magnets themselves. Before a ferromagnetic material is subjected to an external magnetic field, these domains are randomly oriented, resulting in minimal net magnetization of the object as a whole. Imagine a collection of tiny arrows, all pointing in different directions. Their effects mostly cancel each other.
When an external magnetic field is applied, the domains that are already aligned (or nearly aligned) with the external field start to grow, while those that are misaligned shrink. This growth occurs as the domain walls – boundaries between differently oriented domains – move, allowing the favorably aligned domains to expand at the expense of the unfavorably aligned ones. As the external field strength increases, more and more domains align, leading to a stronger overall magnetization of the material.
The ease with which these domain walls move is a crucial factor determining the “softness” or “hardness” of a magnetic material. Soft magnetic materials, like soft iron, have domain walls that move easily, allowing them to magnetize and demagnetize quickly. Hard magnetic materials, like neodymium, have domain walls that are difficult to move, making them retain their magnetism strongly, even after the external field is removed. This “hardness” is essential for creating permanent magnets used in powerful magnetic fasteners.
## The Science of Ferromagnetism: The Key to Powerful Magnets
Ferromagnetism, as described earlier, is the fundamental mechanism behind the strong magnetism observed in materials like iron, nickel, and cobalt. It’s a quantum mechanical phenomenon arising from the exchange interaction between electrons in these materials. This exchange interaction is what encourages the electron spins to align parallel to each other, leading to the formation of magnetic domains. The strength of the exchange interaction, and thus the Curie temperature (the temperature above which ferromagnetism disappears), varies depending on the specific material and its electronic structure.
In addition to the exchange interaction, other factors influence the ferromagnetic behavior of a material. These include the crystal structure of the material, the presence of impurities or defects, and the applied magnetic field. For instance, certain alloys containing rare-earth elements, such as neodymium-iron-boron (NdFeB) alloys, exhibit exceptionally strong ferromagnetism due to their unique crystal structures and electronic configurations. These materials are crucial for creating the powerful permanent magnets used in modern magnetic fasteners.
Understanding ferromagnetism is essential for designing and optimizing magnetic fasteners. By carefully selecting materials and controlling their processing parameters, engineers can tailor the magnetic properties of the fastener to meet specific application requirements. For example, the coercivity (resistance to demagnetization) and remanence (the magnetic field remaining after the magnetizing force is removed) are critical parameters that determine the long-term performance of a magnetic fastener.
## Different Types of Magnets: Selecting the Right Material
The power of a magnetic fastener is heavily dependent on the type of magnet used. There are several types of magnets, each with its own strengths and weaknesses:
* **Permanent Magnets:** These magnets retain their magnetism even after the removal of an external magnetic field. They are ideal for fasteners that require a constant, strong hold. Common types include:
* **Neodymium (NdFeB) Magnets:** The strongest type of permanent magnet, offering exceptional holding power for their size. However, they are also brittle and susceptible to corrosion in humid environments if not properly coated.
* **Samarium Cobalt (SmCo) Magnets:** Known for their high temperature stability and resistance to corrosion, making them suitable for demanding applications. However, they are more expensive and slightly weaker than neodymium magnets.
* **Alnico Magnets:** Composed of aluminum, nickel, and cobalt, alnico magnets boast excellent temperature stability but lower coercivity (resistance to demagnetization) compared to neodymium or samarium cobalt magnets.
* **Ceramic (Ferrite) Magnets:** The most common and cost-effective type of permanent magnet. They are relatively weak but resistant to corrosion and demagnetization.
* **Electromagnets:** These magnets generate a magnetic field only when an electric current flows through them. They consist of a coil of wire wound around a ferromagnetic core (typically iron). The strength of the magnetic field is proportional to the current flowing through the coil and the number of turns in the coil. Electromagnets are often used in applications where adjustable or temporary magnetic force is needed, but consume power to operate.
The choice of magnet material depends on the specific application requirements, including the required holding force, operating temperature, environmental conditions, and cost constraints. For instance, neodymium magnets are often chosen for applications requiring maximum holding power in a compact size, while ceramic magnets may be sufficient for less demanding applications where cost is a primary concern.
## Factors Influencing Magnetic Strength: From Shape to Spacing
Several factors contribute to the overall strength of a magnetic fastener. These factors can be broadly categorized as material properties, geometric design, and external influences:
* **Magnet Material:** As previously discussed, the type of magnet material plays a crucial role. Neodymium magnets will generally offer a much stronger hold than ceramic magnets of the same size and shape. The remanence and coercivity of the magnet material directly impact the strength of the magnetic field it generates.
* **Magnet Shape and Size:** The shape and size of the magnet significantly affect its holding power. A larger magnet, logically, will generally exert a stronger force. However, the shape also matters. A disc-shaped magnet will often have a stronger holding force perpendicular to its surface compared to a rod-shaped magnet of the same volume. The orientation of the magnet’s poles relative to the target material is also critical.
* **Air Gap:** The presence of an air gap between the magnet and the target material dramatically reduces the magnetic force. Even a small air gap can significantly decrease the holding power. This is because air has a high magnetic reluctance (resistance to magnetic flux), hindering the flow of magnetic lines of force. Therefore, minimizing the air gap is crucial for maximizing the fastener’s strength. This can be achieved by ensuring that the surfaces are clean, flat, and in direct contact.
* **Target Material:** The type of material the magnet is attracted to also plays a significant role. Ferromagnetic materials, like steel and iron, provide the best attraction. The permeability of the target material, which measures its ability to concentrate magnetic flux lines, affects the strength of the magnetic force.
* **Temperature:** Temperature can affect the strength of a magnet, especially permanent magnets. As the temperature increases, the magnetic domains can become more disordered, leading to a decrease in magnetization. Different magnet materials have different Curie temperatures (the temperature above which they lose their magnetism).
## Maximizing Holding Power: Design and Optimization Techniques
Optimizing the design of a magnetic fastener involves carefully considering the factors that influence its strength and implementing techniques to maximize its holding power. Some key design and optimization techniques include:
* **Flux Concentration:** Designing the fastener to concentrate the magnetic flux lines in the area of contact with the target material can significantly enhance the holding power. This can be achieved by using strategically shaped pole pieces or ferromagnetic cores to guide and focus the magnetic field.
* **Shielding:** In some applications, it may be necessary to shield the magnetic field to prevent interference with sensitive electronic equipment. This can be achieved by using a ferromagnetic material to enclose the magnet and redirect the magnetic flux lines.
* **Combining Magnets:** For applications requiring exceptionally high holding power, multiple magnets can be used in combination. The magnets can be arranged in various configurations, such as parallel or Halbach arrays, to enhance the overall magnetic field strength and holding force.
* **Surface Preparation:** Ensuring that the surfaces of both the magnet and the target material are clean, flat, and free of contaminants is essential for minimizing the air gap and maximizing the contact area.
* **Coatings:** Applying a protective coating to the magnet can prevent corrosion and improve its durability, especially in harsh environments. Common coatings include nickel, epoxy, and parylene.
* **Finite Element Analysis (FEA):** Employing FEA software can simulate the magnetic field distribution and predict the holding power of a magnetic fastener design. This allows engineers to optimize the design and identify potential weaknesses before fabrication.
## Applications Across Industries: Where Magnetic Fasteners Excel
Magnetic fasteners have found widespread applications across various industries due to their versatility, ease of use, and strong holding power. Some notable examples include:
* **Automotive:** Used in closure systems, sensors, and electric motors. Magnetic latches in glove boxes and interior panels, sensors for position and speed measurements, and powerful permanent magnets in electric traction motors are examples.
* **Aerospace:** Used in aircraft interiors, access panels, and attachment of non-structural components. Their lightweight and secure fastening capabilities are highly valued.
* **Electronics:** Used in speaker systems, hard drives, and electronic enclosures. They offer a secure and easily detachable method of fastening components.
* **Medical:** Used in MRI machines, surgical instruments, and prosthetic devices. High-strength magnets are crucial for advanced medical imaging and precision surgical tools.
* **Manufacturing:** Used in assembly lines, jigs and fixtures, and material handling systems. Magnetic grippers and holding devices improve efficiency and precision in manufacturing processes.
* **Construction:** Used in temporary signage, attachment of decorative elements, and securing building materials. Their ease of installation and removal makes them ideal for temporary applications.
* **Renewable Energy:** Magnetic couplings find extensive use in wind turbine generators, and magnetic levitation finds application in Maglev (Magnetic Levitation) trains.
## The Future of Magnetic Fastening: Innovations and Advancements
The field of magnetic fastening is continuously evolving, with ongoing research and development efforts focused on improving magnet materials, enhancing fastener designs, and expanding their application range. Some promising areas of innovation include:
* **Advanced Magnet Materials:** Research is underway to develop new magnet materials with even higher energy products (a measure of magnet strength) and improved temperature stability. This includes exploring rare-earth-free magnets and advanced alloy compositions.
* **Smart Magnetic Fasteners:** Integrating sensors and microelectronics into magnetic fasteners to enable real-time monitoring of their performance and condition. This could allow for predictive maintenance and improved safety.
* **Tunable Magnetic Fasteners:** Developing fasteners with adjustable magnetic force, allowing for greater flexibility and adaptability in various applications. This could involve using electromagnets or mechanically adjustable magnet configurations.
* **3D-Printed Magnets:** Investigating the use of 3D printing techniques to create magnets with complex shapes and customized magnetic properties. This could enable the fabrication of highly optimized magnetic fasteners for specific applications.
* **Biomagnetic Applications:** Exploring the use of magnetic nanoparticles and micro-robots for targeted drug delivery, medical imaging, and other biomedical applications. These tiny magnetic devices could revolutionize medical diagnostics and treatments.
**Συμπεράσματα**
Magnetic fasteners are far more than simple convenience items. They are a testament to the powerful interplay of atomic physics, materials science, and engineering design. Their surprising strength stems from the fundamental principles of magnetism, the careful selection and processing of magnetic materials, and the optimization of fastener geometry. From holding your refrigerator door closed to powering high-tech medical equipment, magnetic fasteners play a crucial role in numerous aspects of modern life. As research and development continue to push the boundaries of magnet technology, we can expect even more innovative and sophisticated applications of magnetic fasteners in the future. Understanding the science behind these ubiquitous devices allows us to appreciate their ingenuity and unlock their potential for even greater advancements.
## FAQ
### What is the strongest type of magnet for a fastener?
Neodymium (NdFeB) magnets are generally the strongest type of permanent magnet available for fasteners. They offer the highest holding power for their size and weight. However, consider their brittleness and susceptibility to corrosion when selecting them for an application.
### How does an air gap affect the strength of a magnetic fastener?
An air gap significantly reduces the strength of a magnetic fastener. Air has high magnetic reluctance, impeding the flow of magnetic flux lines. Even a small air gap can drastically decrease the holding power. Minimize the air gap for maximum holding strength.
### Are magnetic fasteners suitable for high-temperature applications?
It depends on the type of magnet. Neodymium magnets are susceptible to performance degradation at higher temperatures. Samarium Cobalt (SmCo) magnets offer better temperature stability and are more suitable for high-temperature applications. Consult the magnet’s datasheet for its operating temperature range.
### Can magnetic fasteners be used outdoors?
Yes, but the magnet should be protected from corrosion, which can weaken it over time. Coatings like nickel, epoxy, or parylene can protect the magnet from moisture and other environmental factors. Stainless steel enclosure can further prevent corrosion.
### How do you measure the strength of a magnetic fastener?
The strength of a magnetic fastener is typically measured in terms of its holding force, which is the amount of force required to separate the magnet from a ferromagnetic surface. This can be measured using a pull-off force gauge or a tensile testing machine.
### Are magnetic fasteners permanent?
Permanent magnets, such as neodymium, alnico, samarium cobalt, and ceramic magnets, will retain their magnetism for a very long time under normal conditions. However, exposure to high temperatures, strong opposing magnetic fields, or physical damage can cause them to demagnetize over time.
### Are there any safety concerns with using strong magnetic fasteners?
Strong magnetic fasteners can pose safety risks, particularly to individuals with pacemakers or other implanted medical devices. The strong magnetic field can interfere with the operation of these devices. Additionally, strong magnets can attract ferromagnetic objects with considerable force, potentially causing injury. Always exercise caution and follow safety guidelines when handling strong magnetic fasteners.
### Can I increase the strength of my existing magnetic fastener?
Possibly. Ensuring a clean, flat contact surface between the magnet and the target material to minimize any air gap will help. Concentrating the force onto a specific surface will also help. However, to significantly improve magnetic force, the magnet itself will need to be larger or of a more powerful material.
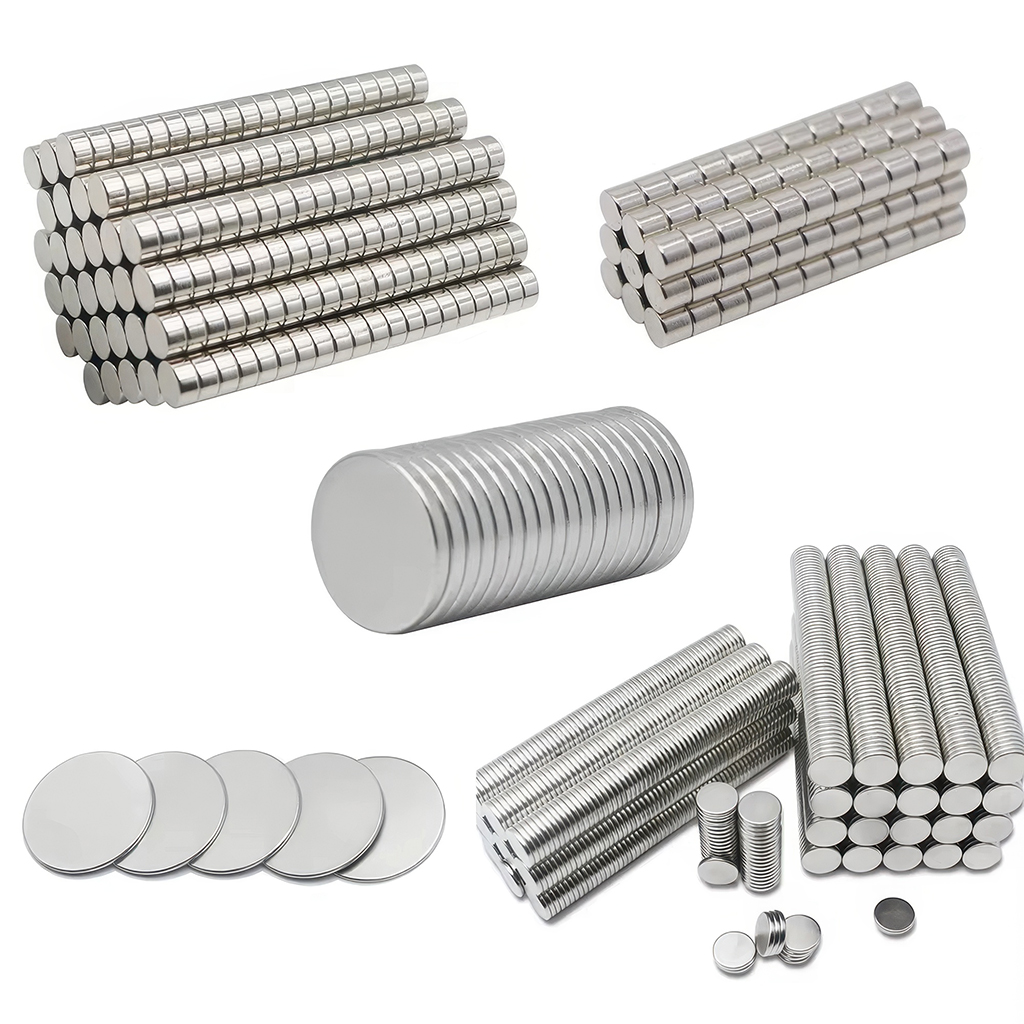