# High-Powered Magnet Breaks Record, Pushing the Boundaries of Field Strength
**Summary:** This article delves into the groundbreaking achievement of a newly developed high-powered magnet that has shattered previous records for magnetic field strength. We explore the science behind this technological marvel, its potential applications across various scientific disciplines and industries, and the implications of this advancement for future research and innovation. Discover how this powerful magnet is poised to revolutionize fields ranging from medicine and materials science to energy and fundamental physics.
## The Quest for Ever Stronger Magnetic Fields
For decades, scientists and engineers have relentlessly pursued the creation of increasingly powerful magnets. The driving force behind this ambition lies in the immense potential of strong magnetic fields to unlock new frontiers in scientific discovery and technological innovation. From probing the fundamental properties of matter to enabling advanced medical imaging and optimizing energy production, the applications of high-field magnets are vast and transformative. This quest is not merely about breaking records; it’s about pushing the boundaries of our understanding of the universe and developing technologies that can address some of humanity’s most pressing challenges.
The development of high-field magnets is a complex and multidisciplinary endeavor, requiring expertise in materials science, electrical engineering, cryogenics, and more. Overcoming the inherent limitations of materials and engineering challenges associated with containing and controlling such immense forces necessitates constant innovation in design, fabrication, and operational techniques. The race to achieve ever-greater field strengths is a testament to human ingenuity and the unwavering pursuit of scientific advancement.
## The Newly Crowned King: A Record-Breaking Achievement
This recently developed magnet represents a significant leap forward in magnet technology, surpassing all previous benchmarks for magnetic field strength. Its creation involved years of research, development, and meticulous engineering. The precise details of its design and composition are closely guarded by the research team, but they have revealed some key aspects of its groundbreaking approach. Using advanced materials and innovative cooling techniques, the team was able to overcome limitations that had previously constrained the performance of high-field magnets.
The successful testing and validation of this magnet mark a major milestone in the field. The measured magnetic field strength significantly exceeds that of any previously existing magnet, opening up new possibilities for experimentation and technological development. The achievement has been celebrated throughout the scientific community as a testament to the power of collaborative research and the relentless pursuit of innovation. This magnet is not just a record-breaker; it’s a game-changer.
## Advanced Materials at the Heart of the Innovation
The record-breaking performance of this magnet is inextricably linked to the development and utilization of advanced materials. Traditional magnet materials, such as iron and alnico, have inherent limitations in terms of the maximum field strength they can sustain. To overcome these limitations, researchers turned to more exotic and technologically challenging materials, including specific alloys of niobium, titanium, and tin, as well as compounds based on rare earth elements. These materials exhibit superior magnetic properties, allowing them to generate and withstand much stronger fields.
The fabrication of these advanced magnet materials is a complex process, often requiring specialized techniques such as powder metallurgy, wire drawing, and thin-film deposition. Furthermore, the materials must be precisely engineered to optimize their magnetic properties and ensure their structural integrity under extreme conditions. The successful integration of these advanced materials into the magnet design was crucial to achieving its record-breaking performance. The right material choice and processing allows for superconducting properties, enabling the flow of massive electrical current that generates extremely strong magnetic fields.
## Cooling Strategies: Maintaining Superconductivity
One of the most critical challenges in building high-field magnets is managing the immense heat generated by the electrical currents flowing through the magnet coils. In conventional magnets, this heat can quickly become prohibitive, limiting the maximum achievable field strength. Superconducting magnets offer a solution to this problem, as they can conduct electricity with virtually no resistance, eliminating heat generation. However, superconductivity only occurs at extremely low temperatures, typically near absolute zero.
Therefore, advanced cooling systems are essential for maintaining the superconductivity of the magnet coils. These systems often involve the use of liquid helium, a cryogenic fluid that can cool materials to temperatures below 4 Kelvin (-269 degrees Celsius). Sophisticated heat exchangers and thermal insulation techniques are employed to minimize heat leaks and ensure efficient cooling. Without these advanced cooling strategies, the record-breaking performance of the magnet would not be possible. Innovative advancements in cryogenics and thermal management are crucial to the design and operation of such magnets.
## Potential Applications in Medical Imaging
The development of this high-powered magnet holds enormous promise for advancing medical imaging techniques. Magnetic Resonance Imaging (MRI) relies on strong magnetic fields to generate detailed images of the human body, allowing doctors to diagnose a wide range of medical conditions. Higher field strength MRI scanners can produce images with higher resolution and greater clarity, enabling the detection of smaller lesions and subtle abnormalities.
The increased sensitivity of high-field MRI could revolutionize the diagnosis and treatment of diseases such as cancer, Alzheimer’s disease, and multiple sclerosis. It could also improve the accuracy of surgical planning and guidance, leading to better patient outcomes. While high-field MRI scanners are already in use, the record-breaking magnet has the potential to push the boundaries of what is possible, offering unprecedented levels of detail and diagnostic capabilities. Its improvements could lead to earlier and more accurate diagnoses, ultimately improving patient care and treatment plans.
## Unlocking New Possibilities in Materials Science
Beyond medical imaging, the high-powered magnet also has significant implications for materials science research. Exposing materials to strong magnetic fields can alter their electronic and magnetic properties, leading to new insights into their fundamental behavior. This knowledge can be used to design and develop novel materials with enhanced properties for a wide range of applications, including energy storage, electronics, and structural materials.
For example, strong magnetic fields can be used to manipulate the alignment of atoms and molecules within a material, creating new crystalline structures or inducing phase transitions. This could lead to the development of materials with enhanced strength, conductivity, or magnetic properties. The high-powered magnet will provide researchers with an unparalleled tool for exploring the behavior of materials under extreme conditions, opening up new avenues for scientific discovery and technological innovation. It allows scientists to see how matter behaves under conditions never replicated before.
## Advancing Fusion Energy Research
One of the most ambitious applications of strong magnetic fields is in the pursuit of fusion energy. Fusion, the process that powers the sun, involves fusing light atomic nuclei together to release tremendous amounts of energy. Achieving sustained fusion on Earth requires confining a superheated plasma at extremely high temperatures and pressures. Magnetic confinement is one of the leading approaches to achieve this, using strong magnetic fields to contain the plasma and prevent it from contacting the walls of the reactor.
Stronger magnetic fields are crucial for improving the efficiency and stability of fusion reactors. They allow for better confinement of the plasma, reducing energy losses and increasing the likelihood of achieving sustained fusion. While fusion energy research is still in its early stages, the development of this high-powered magnet represents a significant step towards realizing the potential of fusion as a clean and sustainable energy source. Better confinement means less energy wasted and higher efficiency, bringing us closer to safe fusion power.
## Exploring Fundamental Physics with Extreme Magnetic Fields
Beyond practical applications, this record-breaking magnet will also serve as a powerful tool for fundamental physics research. Strong magnetic fields can be used to probe the fundamental laws of nature, testing our understanding of quantum mechanics, electromagnetism, and particle physics. For instance, scientists can use strong magnetic fields to study the behavior of exotic particles and materials, such as topological insulators and quantum spin liquids.
These experiments can provide insights into the fundamental nature of matter and the forces that govern the universe. The high-powered magnet will allow researchers to create and study conditions that are impossible to achieve in conventional laboratories, opening up new frontiers in fundamental physics research. Examples are the manipulation and observation of subatomic particles to test theories and observe never-before-seen interactions.
## The Future of High-Field Magnet Technology
The development of this record-breaking magnet is not an end in itself, but rather a stepping stone towards even more powerful and sophisticated magnet technologies. Researchers are already exploring new materials, designs, and cooling techniques that could potentially lead to even higher field strengths in the future. One promising area of research is the development of high-temperature superconductors, which can maintain superconductivity at relatively high temperatures, reducing the need for expensive and complex cryogenic cooling systems.
Another area of ongoing research is the development of resistive magnets powered by high-voltage generators capable of brief pulses of extreme power, generating extremely strong magnetic fields for short durations. Scientists are steadily pushing the boundaries of what is possible, driven by the immense potential of high-field magnets to revolutionize science and technology. The continuous pursuit of innovation in magnet technology will undoubtedly lead to even more groundbreaking discoveries in the years to come.
## Conclusion
The creation of this high-powered magnet, achieving a record-breaking magnetic field strength, is a monumental accomplishment with far-reaching implications. This achievement unlocks new possibilities across diverse fields, including medicine, materials science, energy, and fundamental physics. From enhancing medical imaging resolution and enabling the development of novel materials to advancing fusion energy research and testing fundamental laws of nature, the potential applications are vast and transformative. This breakthrough exemplifies human ingenuity and the relentless pursuit of scientific advancement, paving the way for future innovations in magnet technology and countless discoveries yet to come. The world will be forever altered with the use of this powerful magnet.
## FAQ
### How does a magnet generate a magnetic field?
A magnet generates a magnetic field due to the alignment of the magnetic moments of its constituent atoms. In most materials, these magnetic moments are randomly oriented, cancelling each other out. However, in ferromagnetic materials (like iron, nickel, and cobalt), these moments can spontaneously align, creating a net magnetic field. Electromagnets use electrical currents passing through a coil of wire to create magnetic fields. The higher the current, the stronger the field.
### What is magnetic field strength measured in?
Magnetic field strength is typically measured in Tesla (T) or Gauss (G). 1 Tesla is equal to 10,000 Gauss. The Earth’s magnetic field is about 0.00005 Tesla (0.5 Gauss), while a strong refrigerator magnet might have a field strength of around 0.01 Tesla (100 Gauss). MRI machines often use magnets with field strengths of 1.5 to 7 Tesla. The record-breaking magnet significantly surpasses these numbers, creating a far stronger field.
### What are the main challenges in building high-field magnets?
The main challenges in building high-field magnets include:
* **Material limitations:** Traditional magnet materials have inherent limits to the field strength they can sustain.
* **Heat generation:** The electrical currents flowing through magnet coils generate immense heat, which can degrade performance and even damage the magnet.
* **Mechanical stresses:** The strong magnetic fields exert tremendous mechanical forces on the magnet structure, requiring robust designs to prevent failure.
* **Cryogenics:** Superconducting magnets require extremely low temperatures, necessitating complex and expensive cooling systems.
### What are the benefits of using superconducting magnets?
Superconducting magnets offer several benefits over conventional magnets:
* **Zero resistance:** Superconductors conduct electricity with virtually no resistance, eliminating heat generation and allowing for higher current densities.
* **Stronger fields:** Superconducting magnets can generate much stronger magnetic fields than conventional magnets.
* **Energy efficiency:** Superconducting magnets consume less energy than conventional magnets, making them more cost-effective to operate.
### How are strong magnetic fields used in particle accelerators?
Strong magnetic fields are crucial for guiding and focusing beams of particles in particle accelerators. These fields bend the paths of charged particles, keeping them moving in a circular or spiral trajectory. The stronger the magnetic field, the higher the energy the particles can achieve. Particle accelerators use high-field magnets to collide beams of particles at near the speed of light, allowing scientists to probe the fundamental constituents of matter and the forces that govern the universe.
### Are there any safety concerns associated with strong magnetic fields?
Yes, strong magnetic fields can pose safety risks. They can interfere with electronic devices, such as pacemakers and other medical implants. They can also attract ferromagnetic objects, such as tools and equipment, with considerable force, potentially causing injury or damage. It is important to follow strict safety protocols when working near high-field magnets, including removing all metallic objects and restricting access to authorized personnel.
### What is the potential impact of this magnet on fusion power development?
The increased magnetic field strength enables better plasma confinement, which is crucial for achieving sustained fusion reactions. Stronger magnetic fields reduce energy leaks from the plasma, which are a major obstacle in developing fusion reactors. This magnet represents a significant advancement towards making fusion energy a viable and sustainable energy source.
### Why is it so difficult to increase the strength of magnets?
Increasing magnetic field strength comes with several challenges. The materials must withstand immense magnetic forces, requiring advanced material science. Cooling becomes increasingly difficult as field strength rises, necessitating advanced cryogenic systems. Electrical current is greatly increased which requires specialized conductors. Each factor puts tremendous strain on designs and engineering prowess. Overcoming one challenge often exacerbates another.
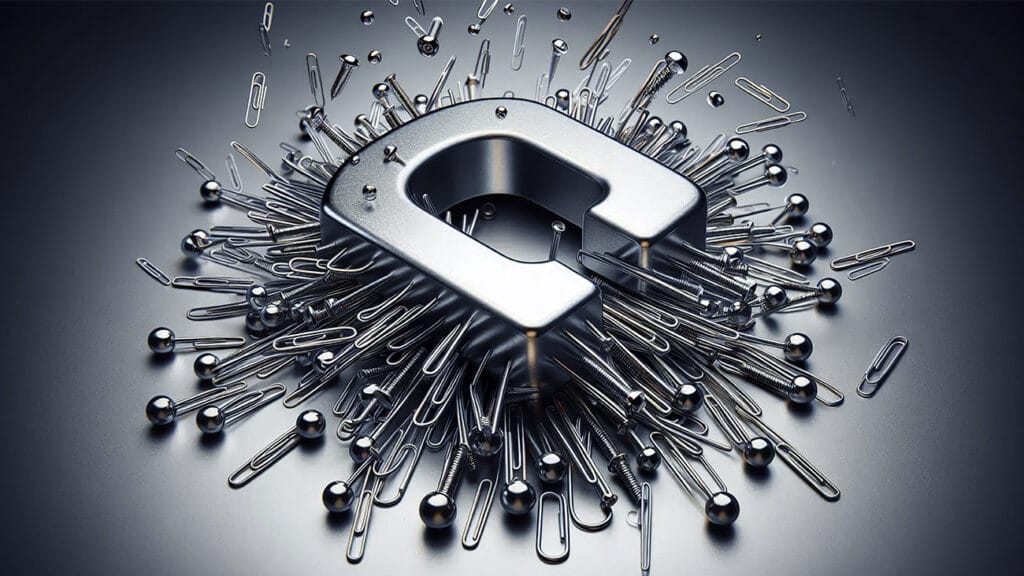