# Magnetic Materials Explained: A Deep Dive from Basic Compounds to Advanced Alloys
This article provides a comprehensive exploration of magnetic materials, covering the fundamental properties of magnetic compounds and the cutting-edge developments in advanced magnetic alloys. Whether you’re a student, engineer, or simply curious about the science behind magnets, this guide will unravel the complexities of magnetism and its diverse applications. Learn about the different types of magnetic materials, their underlying atomic structure, and how these properties are harnessed in various technologies.
## Understanding the Fundamentals of Magnetism
At its core, magnetism arises from the movement of electric charges within atoms. These moving charges, primarily electrons orbiting the nucleus and spinning on their axis, generate tiny magnetic fields. A material’s overall magnetic behavior depends on how these individual atomic magnetic moments align. In some materials, these moments are randomly oriented, resulting in no net magnetic field. In others, the moments tend to align, leading to observable magnetic properties.
The strength of a magnetic field is typically measured in units called Tesla (T) or Gauss (G) (1 T = 10,000 G). The magnetic field strength is crucial for determining the suitability of a material for different applications, ranging from magnetic recording to medical imaging and power generation. Key concepts to grasp are magnetic susceptibility, which measures how easily a material becomes magnetized in an external field, and magnetic permeability, which indicates how well a material allows magnetic flux to pass through it.
## Classifying Magnetic Materials: Diamagnetism, Paramagnetism, and Ferromagnetism
Magnetic materials are broadly classified into three main categories based on their response to an external magnetic field: diamagnetic, paramagnetic, and ferromagnetic. Diamagnetic materials exhibit a weak repulsion to an external magnetic field. This occurs because the applied field induces a small opposing magnetic moment in the atoms. Examples include copper, gold, and water.
Paramagnetic materials, on the other hand, are weakly attracted to an external magnetic field. This attraction arises from the partial alignment of existing atomic magnetic moments with the applied field. Unlike diamagnetism, paramagnetism is temperature-dependent, with the attraction decreasing as temperature increases due to the increased thermal agitation disrupting the alignment. Examples of paramagnetic materials include aluminum, titanium, and platinum.
Ferromagnetic materials are strongly attracted to an external magnetic field and can retain magnetization even after the field is removed. This is due to the spontaneous alignment of atomic magnetic moments within regions called magnetic domains. Below a certain temperature called the Curie temperature, these domains remain aligned, leading to the material’s strong magnetic behavior. Examples include iron, nickel, and cobalt.
## Diving Deeper: Antiferromagnetism and Ferrimagnetism
Beyond the three primary categories, antiferromagnetism and ferrimagnetism offer more complex magnetic behaviors. Antiferromagnetic materials also exhibit ordered magnetic moments, but instead of aligning parallel to each other as in ferromagnetism, they align antiparallel. This antiparallel alignment results in a near-zero net magnetic moment at the macroscopic level, even though the individual atoms possess significant magnetic moments. Examples include chromium and manganese oxide (MnO).
Ferrimagnetic materials share similarities with both ferromagnetism and antiferromagnetism. They exhibit antiparallel alignment of magnetic moments, but the magnitudes of these moments are unequal. This unequal cancellation leads to a large net magnetic moment, similar to ferromagnets. Ferrites, which are ceramic materials containing iron oxide mixed with other metallic oxides, are common examples of ferrimagnetic materials.
## Exploring Ferromagnetic Materials: Hysteresis and Domain Theory
Ferromagnetic materials are characterized by their ability to retain magnetization after the external field is removed, an effect known as remanence. This phenomenon is represented by the material’s hysteresis loop, a graph plotting the magnetic field strength (H) against the magnetization (M). The hysteresis loop provides valuable information about a material’s coercivity (the field required to demagnetize the material) and its saturation magnetization (the maximum magnetization it can achieve).
The domain theory explains the microscopic origin of ferromagnetism. A ferromagnetic material consists of numerous small regions called magnetic domains, each with its own uniform magnetization direction. In an unmagnetized state, these domains are randomly oriented, resulting in zero net magnetization. When an external magnetic field is applied, the domains aligned with the field grow at the expense of the others, leading to a net magnetization of the material. Upon removal of the field, some domains remain aligned, resulting in remanence.
## The Importance of Magnetic Anisotropy
Magnetic anisotropy refers to the tendency of a material’s magnetization to align along specific crystallographic directions. This preferred direction is called the easy axis, while directions that are harder to magnetize are called hard axes. Anisotropy can be caused by various factors, including the crystal structure of the material (magnetocrystalline anisotropy), the shape of the material (shape anisotropy), and the presence of internal stresses (stress anisotropy).
Manipulating magnetic anisotropy is crucial for tailoring the properties of magnetic materials for specific applications. For example, in magnetic recording media, a high degree of anisotropy is desired to ensure that the recorded information is stable and resistant to thermal fluctuations. In contrast, some applications, such as soft magnetic materials used in transformers, require low anisotropy to minimize losses due to domain wall motion.
## Soft Magnetic Materials: Properties and Applications
Soft magnetic materials are characterized by their high permeability, low coercivity, and low remanence. These properties allow them to be easily magnetized and demagnetized, making them ideal for applications where the magnetic field needs to be rapidly switched or modulated. Examples of soft magnetic materials include iron-silicon alloys, nickel-iron alloys (permalloy), and amorphous alloys.
Soft magnetic materials are widely used in transformers, inductors, motors, and other electromagnetic devices. Their high permeability allows them to efficiently channel magnetic flux, reducing energy losses and improving device performance. In transformers, soft magnetic cores minimize hysteresis losses, while in motors, they help to concentrate the magnetic field in the air gap, increasing torque and efficiency.
## Hard Magnetic Materials: Permanent Magnets and Their Uses
Hard magnetic materials, also known as permanent magnets, possess high coercivity, high remanence, and high energy product (a measure of the magnet’s ability to store magnetic energy). These properties allow them to retain their magnetization even in the presence of strong opposing fields, making them ideal for applications requiring a stable and strong magnetic field. Examples of hard magnetic materials include alnico alloys, ferrite magnets, rare-earth magnets (such as neodymium magnets and samarium-cobalt magnets).
Permanent magnets are ubiquitous in modern technology, found in applications ranging from loudspeakers and electric motors to magnetic resonance imaging (MRI) machines and computer hard drives. Neodymium magnets, in particular, are known for their exceptional strength and are used in high-performance motors and generators, as well as in energy-efficient devices. Ferrite magnets, while weaker than neodymium magnets, are cost-effective and are used in a wide range of applications, including refrigerator magnets and small electric motors.
## Advanced Magnetic Alloys: Innovations and Future Trends
Ongoing research is focused on developing advanced magnetic alloys with enhanced properties, such as higher saturation magnetization, higher Curie temperature, and improved corrosion resistance. Nanocrystalline alloys, which consist of ultrafine grains with sizes in the nanometer range, offer superior soft magnetic properties compared to their conventional counterparts. Heusler alloys, a class of intermetallic compounds, exhibit a wide range of magnetic and electronic properties, including half-metallicity and shape memory effects, making them promising for spintronic devices and other advanced applications.
The future of magnetic materials research is likely to focus on the development of new materials with tailored properties for specific applications, as well as on the exploration of novel phenomena, such as topological magnetism and skyrmions. These advancements hold the potential to revolutionize various fields, including energy, information technology, and medicine.
## Conclusion
From the fundamental principles of atomic magnetism to the sophisticated design of advanced alloys, magnetic materials play a crucial role in modern technology. Understanding the different types of magnetic materials, their properties, and their applications is essential for anyone working in the fields of engineering, physics, or materials science. This article provided an overview of the key concepts and advancements in the field, highlighting the importance of ongoing research and development in pushing the boundaries of magnetic material science. As technology continues to evolve, magnetic materials will undoubtedly continue to be at the forefront of innovation, enabling new and exciting possibilities.
## FAQ
### Why are some materials magnetic and others not?
The magnetic properties of a material depend on the alignment of the magnetic moments of its constituent atoms. In non-magnetic materials, these moments are randomly oriented, cancelling each other out. In magnetic materials, the moments tend to align, resulting in a net magnetic field. The type of alignment (parallel, antiparallel, etc.) determines the specific type of magnetism exhibited by the material.
### What is the difference between soft and hard magnets?
Soft magnets are easily magnetized and demagnetized, making them suitable for applications that require rapidly changing magnetic fields. Hard magnets, also known as permanent magnets, retain their magnetization even after the external field is removed, making them ideal for applications requiring a stable magnetic field.
### What are some common applications of magnetic materials?
Magnetic materials are used in a wide range of applications, including electric motors, generators, transformers, hard drives, loudspeakers, medical imaging equipment (MRI), microwave devices and magnetic shielding. The specific material used depends on the application’s requirements for magnetic strength, stability, and operating frequency.
### What is a hysteresis loop and what information does it provide?
A hysteresis loop is a graph that plots the magnetic field strength (H) against the magnetization (M) of a ferromagnetic material. It provides information about the material’s coercivity, remanence, and saturation magnetization, which are important parameters for characterizing its magnetic properties.
### What are rare-earth magnets and why are they so strong?
Rare-earth magnets, such as neodymium magnets and samarium-cobalt magnets, are a type of permanent magnet containing rare-earth elements. They are known for their exceptional strength due to their high saturation magnetization and high coercivity, which arise from the unique electronic structure of the rare-earth elements.
### What are some areas of ongoing research in magnetic materials?
Current research efforts in magnetic materials focus on developing new alloys with enhanced properties, such as higher saturation magnetization, higher Curie temperature, and improved corrosion resistance. Research is also being conducted on novel magnetic phenomena, such as topological magnetism and skyrmions, which hold potential for future technological applications.
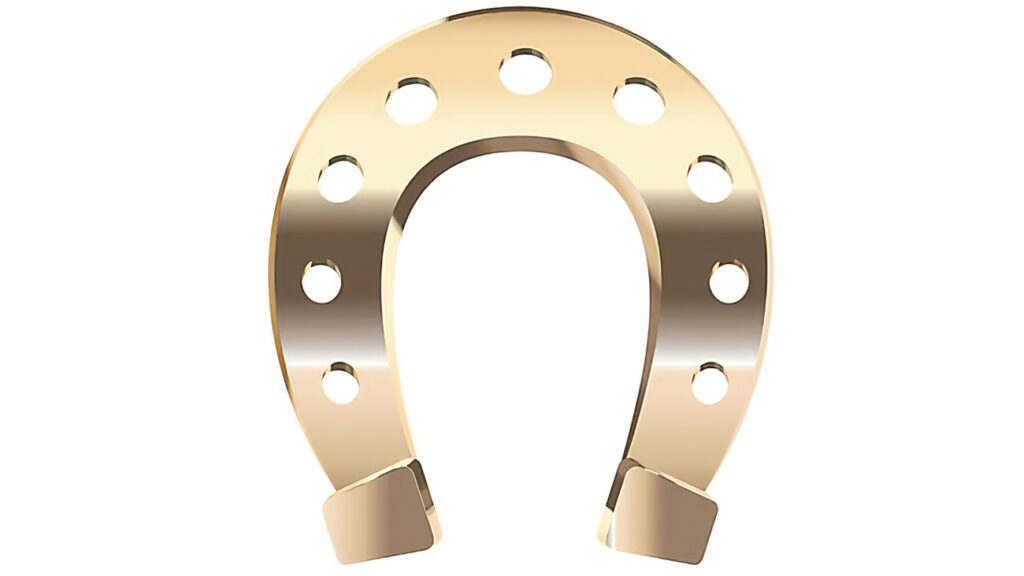