# The Unseen Force: A Deep Dive into the Physics Behind Magnetic Fastener Holding Power
**Summary:** Ever wondered how those sleek magnetic closures on your laptop or that powerful magnet holding tools in your garage work? This comprehensive guide unravels the science behind magnetic fasteners, exploring the fundamental physics governing their holding power. From atomic magnetism to real-world applications and limitations, you’ll gain a deep understanding of these ubiquitous but often misunderstood components. Learn how different materials, magnet shapes, and external factors influence their performance, and discover the key considerations for selecting the right magnetic fastener for your needs.
## Understanding the Basics: What is Magnetism?
Magnetism, at its core, is a fundamental force of nature arising from the movement of electric charges. Within atoms, electrons continuously orbit the nucleus and spin on their axis, creating tiny magnetic fields. In most materials, these fields are randomly oriented, effectively canceling each other out. However, in certain materials like iron, nickel, and cobalt, known as ferromagnetic materials, the electron spins tend to align spontaneously within small regions called magnetic domains.
When an external magnetic field is applied, these domains align with the field, resulting in a strong, net magnetic moment for the entire material. This alignment is what gives magnets their attractive and repulsive properties. The strength of the magnetic field is measured in Tesla (T) or Gauss (G) (1 Tesla = 10,000 Gauss). The higher the value, the stronger the magnetic field and the greater the potential holding power for a magnetic fastener.
The interaction between magnetic fields is governed by the fundamental laws of electromagnetism. Like poles repel, opposite poles attract. This seemingly simple principle forms the basis for all magnetic interactions, whether it’s a small refrigerator magnet or a powerful industrial lifting magnet. The strength of attraction or repulsion decreases rapidly with distance, highlighting the importance of close proximity in magnetic fastener design.
## Diving Deeper: Atomic Origins of Magnetic Moments
To truly grasp the power of magnetic fasteners, we need to delve into the atomic realm where magnetism originates. Electrons, those subatomic particles whizzing around the nucleus, possess an intrinsic property called “spin.” This spin, analogous to a tiny spinning top (although not literally spinning in the classical sense), generates a tiny magnetic dipole moment. Think of it as a microscopic bar magnet.
In most atoms, electrons pair up with opposite spins, effectively canceling out their magnetic moments. However, some atoms, particularly those with unfilled electron shells, have unpaired electrons. These unpaired electrons contribute to a net atomic magnetic moment. The greater the number of unpaired electrons, the stronger the atomic magnetic moment. Elements like iron (Fe) have several unpaired electrons, making them highly susceptible to magnetization.
Furthermore, the orbital motion of electrons also contributes to the atomic magnetic moment. However, in most solids, the orbital contribution is significantly smaller than the spin contribution. The interplay between spin and orbital motion, described by quantum mechanics, dictates the magnetic properties of different materials. Understanding these atomic-level interactions is crucial for designing materials with tailored magnetic properties for specific fastener applications.
## Types of Magnetic Materials: From Alnico to Neodymium
Magnetic materials can be broadly categorized into several types, each with unique properties that influence their suitability for different magnetic fastener applications. Permanent magnets, the workhorses of the magnetic fastener world, retain their magnetization even in the absence of an external magnetic field. The most common types of permanent magnets include:
* **Alnico Magnets:** Composed of aluminum, nickel, and cobalt, alnico magnets are known for their high Curie temperature (the temperature above which they lose their magnetism) and excellent temperature stability. However, they have relatively low coercivity (resistance to demagnetization) compared to other types.
* **Ferrite Magnets:** Also known as ceramic magnets, ferrite magnets are made from iron oxide and other ceramic materials. They are inexpensive, corrosion-resistant, and have good coercivity, making them ideal for applications where cost is a primary concern. However, they have relatively low energy product (a measure of magnetic field strength) compared to other types.
* **Samarium Cobalt (SmCo) Magnets:** SmCo magnets offer a good balance of high energy product, high coercivity, and good temperature stability. They are more expensive than ferrite magnets but offer superior performance in demanding applications.
* **Neodymium (NdFeB) Magnets:** Often referred to as “neo” magnets, neodymium magnets are the strongest type of permanent magnet commercially available. They have an exceptionally high energy product, making them ideal for applications where maximum holding power is required. However, they have a lower Curie temperature than alnico and SmCo magnets and are more susceptible to corrosion.
Each magnet type has advantages and disadvantages, and the choice of material depends heavily on the specific application requirements, including required holding power, operating temperature, cost, and size constraints.
## The Magnetic Circuit: Optimizing Flux for Maximum Hold
The performance of a magnetic fastener is not solely determined by the magnet itself but also by the “magnetic circuit” – the path that the magnetic flux (the measure of the magnetic field) takes as it travels from the magnet, through the air gap, into the attracted material (often steel), and back to the magnet. Optimizing this circuit is crucial to maximizing the holding power.
The air gap between the magnet and the attracted material is a critical factor. Air has a very high magnetic reluctance (resistance to magnetic flux), meaning that the magnetic field strength drops off dramatically as the air gap increases. Minimizing the air gap is essential for maximizing the holding power. This is why magnetic fasteners often incorporate steel or other ferromagnetic materials in their design to provide a low-reluctance path for the magnetic flux.
The shape of the magnet and the surrounding steel components also plays a significant role. Concentrating the magnetic flux at the contact surface maximizes the attractive force. This can be achieved through careful design of the magnetic circuit, using techniques such as shaping the magnet and adding pole pieces (ferromagnetic materials that concentrate the flux). Computer simulations are often used to optimize the magnetic circuit design for specific applications.
## Factors Affecting Holding Power: Beyond the Magnet
While the magnet itself is the core component, several factors beyond the material properties of the magnet significantly impact the holding power of a magnetic fastener. These include:
* **Surface Condition:** The cleanliness and flatness of the mating surfaces are crucial. Dust, dirt, paint, or any other contaminants can create an air gap, significantly reducing the holding power. Rough surfaces also reduce the effective contact area, hindering magnetic flux flow.
* **Material of the Attracted Surface:** The type of metal being attracted plays a significant role. Ferromagnetic materials like steel provide a low-reluctance path for the magnetic flux, resulting in strong attraction. Non-ferrous materials like aluminum or brass have little to no magnetic attraction.
* **Thickness of the Attracted Surface:** If the steel is too thin, it can become magnetically saturated, limiting the amount of magnetic flux it can carry. This reduces the holding power. A sufficient thickness of steel is necessary to fully utilize the magnet’s potential.
* **Temperature:** Most magnets lose strength as temperature increases. The Curie temperature is the point at which a magnet loses its magnetism entirely. However, even well below the Curie temperature, the magnetic field strength can decrease significantly with increasing temperature.
* **External Magnetic Fields:** External magnetic fields can either enhance or weaken the holding power, depending on their orientation relative to the fastener’s magnetic field. Strong opposing fields can even demagnetize the fastener over time.
* **Shear vs. Tensile Force:** Magnetic fasteners typically exhibit significantly lower holding power when subjected to shear forces (forces parallel to the surface) compared to tensile forces (forces perpendicular to the surface). This is because shear forces can cause the magnet to slip or break free from the attracted surface.
Understanding these factors is essential for selecting the appropriate magnetic fastener and ensuring reliable performance in a given application.
## Demagnetization: The Silent Threat to Holding Power
Demagnetization, the loss of magnetic strength over time, is a significant concern for magnetic fasteners. Several factors can contribute to demagnetization, including:
* **High Temperatures:** As mentioned earlier, elevated temperatures can reduce the magnetic field strength. Exceeding the Curie temperature will permanently demagnetize the magnet. Even operating below the Curie temperature can lead to irreversible demagnetization over time.
* **Strong Opposing Magnetic Fields:** Exposure to strong opposing magnetic fields can flip the magnetic domains within the magnet, reducing its net magnetic moment. This is particularly problematic for magnets with low coercivity.
* **Mechanical Shock and Vibration:** Repeated mechanical shock and vibration can gradually disorient the magnetic domains, leading to a loss of magnetic strength. This is more pronounced in magnets with a less stable domain structure.
* **Corrosion:** Corrosion products can create an insulating layer on the magnet’s surface, reducing the effective surface area and hindering the flow of magnetic flux. Corrosion can also directly attack the magnetic material, degrading its properties.
Protecting magnets from these demagnetizing influences is crucial for maintaining their holding power and ensuring long-term reliability. This can involve selecting magnets with high coercivity, applying protective coatings, and avoiding exposure to extreme temperatures, strong magnetic fields, and harsh environments.
## Testing and Measuring Holding Power: Ensuring Reliability
Ensuring the reliability of magnetic fasteners requires rigorous testing and measurement of their holding power. Several methods are used to quantify the performance of these components:
* **Pull Test:** This is the most common method for measuring holding power. The magnetic fastener is attached to a steel plate, and a tensile force is applied until the magnet separates from the plate. The force required to break the connection is recorded as the holding power.
* **Shear Test:** Similar to the pull test, but the force is applied parallel to the surface. This test measures the shear strength of the magnetic fastener, which is typically lower than the tensile strength.
* **Gaussmeter Measurement:** A gaussmeter is used to measure the magnetic field strength at the surface of the magnet. This provides an indication of the magnet’s overall magnetic strength.
* **Finite Element Analysis (FEA):** Computer simulations using FEA software can predict the holding power of magnetic fasteners based on their geometry, material properties, and external factors. This allows for design optimization and performance prediction without physical prototyping.
These tests provide valuable data for characterizing the performance of magnetic fasteners, comparing different designs, and ensuring that they meet the required specifications. Regular testing is crucial for maintaining quality control and ensuring the long-term reliability of magnetic fastener systems.
## Applications of Magnetic Fasteners: From Home to Industry
Magnetic fasteners have become ubiquitous in a wide range of applications, spanning from everyday household items to sophisticated industrial equipment. Here are some examples:
* **Cabinet and Door Latches:** Magnetic latches provide a simple and reliable way to keep cabinets and doors closed.
* **Tool Holders:** Magnetic tool holders offer a convenient way to organize and access tools in a garage or workshop.
* **Magnetic Jewelry Clasps:** Small but strong magnets are used to create secure and easy-to-use jewelry clasps.
* **Automotive Sensors:** Magnetic sensors are used in various automotive applications, such as detecting wheel speed and position.
* **Industrial Lifting Magnets:** Powerful lifting magnets are used in factories and construction sites to lift and move heavy metal objects.
* **Medical Devices:** Magnetic fasteners are used in some medical devices, such as prosthetic limbs and surgical instruments.
* **Renewable Energy Systems:** Magnetic bearings are used in some wind turbine generators to reduce friction and improve efficiency.
* **Electronics:** Magnetic fasteners are used in laptops, tablets, and other electronic devices for closures and connections.
The versatility of magnetic fasteners stems from their ease of use, reliability, and ability to provide non-mechanical attachment, making them an attractive alternative to traditional fasteners in many applications. As technology advances, the applications of magnetic fasteners will continue to expand.
## Future Trends: Innovations in Magnetic Fastener Technology
The field of magnetic fasteners is constantly evolving, with ongoing research and development efforts focused on improving performance, reducing cost, and expanding the range of applications. Some of the key trends include:
* **Development of New Magnetic Materials:** Researchers are exploring new materials with higher energy product, higher coercivity, and improved temperature stability. This includes the development of rare-earth-free magnets to reduce dependence on critical materials.
* **Advanced Manufacturing Techniques:** Additive manufacturing (3D printing) is being used to create complex magnet shapes and magnetic circuits that are difficult or impossible to produce using traditional methods.
* **Smart Magnetic Fasteners:** Integrating sensors and electronics into magnetic fasteners allows for real-time monitoring of holding power, temperature, and other parameters. This enables predictive maintenance and improved safety in critical applications.
* **Magnetically Assisted Joining Techniques:** Hybrid joining methods that combine magnets with other techniques, such as welding or adhesive bonding, are being developed to create stronger and more reliable joints.
* **Miniaturization:** Advances in nanotechnology are enabling the creation of smaller and more powerful magnetic fasteners for microscale applications.
These innovations will pave the way for new and exciting applications of magnetic fasteners in the future, further solidifying their role as a crucial component in numerous industries.
## Conclusion
Magnetic fasteners, seemingly simple in their function, rely on a complex interplay of fundamental physics. From the atomic origins of magnetism to the careful design of magnetic circuits and the mitigating of demagnetization effects, a deep understanding of these principles is crucial for selecting and utilizing magnetic fasteners effectively. By considering the type of magnetic material, optimizing the magnetic circuit, and accounting for external factors like temperature and surface conditions, engineers and designers can harness the power of magnetism for a wide range of applications, from everyday conveniences to critical industrial processes. As research continues to push the boundaries of magnetic materials and manufacturing techniques, the future of magnetic fasteners promises even greater performance, versatility, and innovation.
## FAQ
### ### What is the difference between Gauss and Tesla?
Gauss and Tesla are both units of magnetic field strength. Tesla is the SI unit of magnetic flux density, while Gauss is a CGS unit. The conversion factor is 1 Tesla = 10,000 Gauss. Tesla is generally used in scientific and engineering applications, while Gauss is often used in older literature or for less precise measurements.
### ### How do I choose the right magnetic fastener for my application?
Choosing the right magnetic fastener depends on several factors, including the required holding power, operating temperature, size constraints, environmental conditions, and cost. Consider the type of magnetic material (e.g., neodymium, ferrite), the shape and size of the magnet, the design of the magnetic circuit, and the surface condition of the mating materials. Consult with a magnet supplier or specialist for expert advice.
### ### Are neodymium magnets dangerous?
Neodymium magnets are very strong and can pose some risks if not handled properly. The magnets can pinch skin between themselves or attract to metal objects with considerable force, causing injury. Swallowing multiple small magnets can cause serious internal injuries. It’s also important to keep neodymium magnets away from electronic devices and pacemakers, as they can interfere with their operation.
### ### Can magnets lose their strength over time?
Yes, magnets can lose their strength over time due to factors such as high temperatures, exposure to strong opposing magnetic fields, mechanical shock, and corrosion. This is known as demagnetization. The rate of demagnetization depends on the type of magnet, its operating environment, and the severity of the demagnetizing influences.
### ### How can I protect magnets from corrosion?
Protecting magnets from corrosion is essential for maintaining their holding power and extending their lifespan. Common methods include applying protective coatings such as nickel, epoxy, or plastic. Choosing a corrosion-resistant magnet material, such as ferrite or samarium cobalt, is also an effective strategy. Avoiding exposure to harsh chemicals and moisture is also important.
### ### What is the Curie temperature?
The Curie temperature is the temperature above which a ferromagnetic material loses its magnetism. At this temperature, the thermal energy overcomes the magnetic ordering forces, causing the magnetic domains to become randomly oriented. The Curie temperature varies depending on the type of magnetic material.
### ### What is coercivity?
Coercivity is a measure of a magnet’s resistance to demagnetization. It is the amount of opposing magnetic field required to reduce the magnet’s magnetization to zero. Magnets with high coercivity are more resistant to demagnetization from external fields and are therefore more suitable for demanding applications.
### ### Can I increase the holding power of a magnetic fastener?
Yes, there are several ways to increase the holding power of a magnetic fastener. These include using a stronger magnet material, optimizing the magnetic circuit design, minimizing the air gap between the magnet and the attracted material, ensuring clean and flat mating surfaces, and using a thicker steel plate.
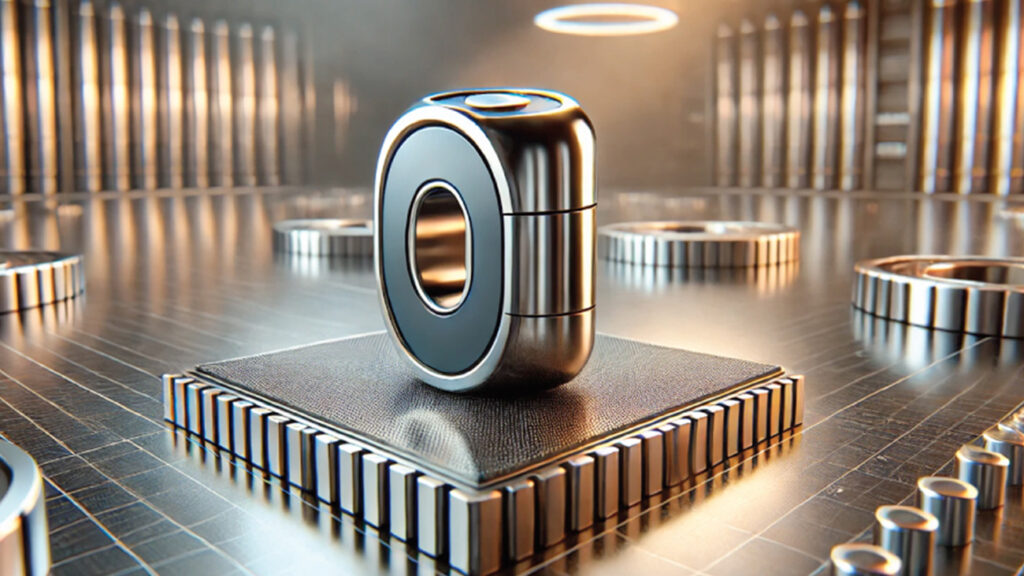