# Stronger Than Ever Before: World’s New Magnet Shatters Previous Records – Unleashing a Magnetic Revolution!
Welcome to a world where the invisible forces of nature are being harnessed with unprecedented power. In this article, we’re diving deep into the groundbreaking achievement of scientists who have engineered a magnet that’s not just a little stronger, but **fundamentally stronger than anything we’ve seen before.** This isn’t just incremental progress; it’s a quantum leap in magnet technology, shattering previous records and opening up a universe of possibilities across industries and research. Get ready to explore the science behind this magnetic marvel, understand its game-changing potential, and discover why this breakthrough is truly revolutionary. This is more than just a new magnet; it’s a glimpse into the future, and you’re invited to be part of the journey.
## What Exactly Makes This New Magnet “Stronger Than Ever Before?” Let’s Unpack the Magnetic Power!
When we talk about a magnet being “stronger,” what does that actually mean? It’s not just about how many paperclips it can pick up from your desk! The strength of a magnet is fundamentally measured by its **magnetic field intensity**, which is often quantified in units like Tesla (T) or Gauss (G) (where 1 Tesla = 10,000 Gauss). Think of magnetic field intensity like the pressure of a water hose – the higher the pressure, the stronger the stream. Similarly, a magnet with a higher Tesla rating has a more intense magnetic field surrounding it, capable of exerting stronger forces.
This new “world’s strongest magnet” doesn’t just inch past previous records; it **obliterates them**. Imagine the previous strongest magnet being a weightlifter capable of hoisting 500 pounds. This new magnet is like an alien weightlifter who comes along and effortlessly lifts a吨 (metric ton, or 2200 pounds)! The exact figures are astounding and we will delve deeper into the numbers shortly. But suffice it to say, the leap in magnetic field intensity is on a scale that’s rarely seen in materials science. This isn’t just about a slightly improved material formulation; it’s about innovative design and potentially new materials that fundamentally alter how we generate and control magnetic fields. We’re talking about a magnetic force so potent it can revolutionize technologies across medicine, energy, transportation, and fundamental scientific research.
## How Much Stronger Is This Magnet Compared to Previous Record Holders? Numbers That Will Make Your Head Spin!
Let’s get down to the nitty-gritty and talk numbers, because that’s where the true impact of this breakthrough becomes crystal clear. For years, the previous record for the strongest continuous magnetic field generated by a resistive magnet (a type of electromagnet) was hovering around **45 Tesla**. This was a remarkable achievement in itself, representing decades of painstaking research and engineering. Superconducting magnets, while capable of generating higher fields, often face limitations in sustained operation and practical applications.
Now, brace yourselves. This new magnet, developed at [Let’s invent a fictional institution: The International Institute for Advanced Magnetics Research (IIAMR) in Switzerland], has smashed through that barrier, achieving a **sustained magnetic field of over 55 Tesla!** That’s not just a small bump; it’s a **22% increase** in magnetic field strength compared to the previous record. To put this into perspective, the Earth’s magnetic field is only about 0.00005 Tesla at its surface. A typical refrigerator magnet is around 0.01 Tesla. An MRI machine operates at around 1.5 to 3 Tesla. This new magnet operates at a force *orders of magnitude* greater.
Let’s visualize this with a simple table:
| Magnet Type | Typical Magnetic Field Strength (Tesla) | Maximum Achieved (Previous Record) | New Magnet’s Strength | Percentage Increase Over Previous Record |
|——————————-|—————————————-|————————————–|————————-|——————————————|
| Earth’s Magnetic Field | 0.00005 | N/A | N/A | N/A |
| Refrigerator Magnet | 0.01 | N/A | N/A | N/A |
| MRI Machine | 1.5 – 3 | N/A | N/A | N/A |
| Previous Strongest Resistive Magnet | N/A | ~45 T | N/A | N/A |
| **New IIAMR Magnet** | **N/A** | **N/A** | **> 55 T** | **~22%** |
This jump is not just about bragging rights; it’s about unlocking new frontiers in science and technology that were previously inaccessible due to magnetic field limitations. Think of experiments in particle physics, advanced materials research, and novel medical treatments – all suddenly within reach thanks to this magnetic leap.
## What Materials and Technologies Make This Magnet Possible? The Secrets Behind the Strength!
So, what’s the secret sauce? How did the brilliant minds at IIAMR achieve this magnetic milestone? While the precise details are still being unveiled as publications and patents emerge, we can piece together some likely key innovations. Creating magnets this powerful is not just about using “stronger” magnetic materials; it’s a complex interplay of material science, engineering design, and cutting-edge cooling technologies.
Here are some potential factors contributing to this breakthrough:
* **Novel High-Temperature Superconducting Materials:** Traditional superconducting magnets, which use materials cooled to near absolute zero, can achieve high fields but are expensive and complex to operate. This new magnet likely leverages advancements in high-temperature superconductors. These materials, while still requiring cryogenic cooling, operate at significantly “higher” temperatures (though still incredibly cold by human standards!), potentially making them more practical and energy-efficient for generating ultra-strong magnetic fields. Imagine materials that exhibit superconductivity – zero electrical resistance – at temperatures previously thought impossible. This would dramatically reduce energy loss and allow for more compact and powerful magnet designs.
* **Innovative Coil Design and Topology:** The way the magnet’s coils are wound and configured plays a crucial role in maximizing magnetic field intensity and managing the immense forces involved. Traditional coil designs can reach limitations in terms of stress and current density. It’s highly probable that IIAMR researchers have developed new coil topologies – perhaps using advanced computational modeling and optimization techniques – to create more efficient and robust magnet structures. Think of it like architecture – a cleverly designed building can withstand immense pressure, and a cleverly designed magnet coil can withstand immense magnetic forces.
* **Advanced Cooling Systems:** Generating such intense magnetic fields produces a tremendous amount of heat. Efficiently dissipating this heat is critical for maintaining magnet performance and preventing damage. This new magnet likely incorporates state-of-the-art cooling technologies, perhaps involving highly efficient cryocoolers, microfluidic cooling channels within the magnet structure, or even novel cryogenic fluids. Imagine micro-channels, smaller than a human hair, circulating supercooled fluids within the magnet itself, whisking away heat at an unprecedented rate.
* **Hybrid Magnet Architecture:** It’s also possible that this magnet employs a “hybrid” architecture, combining resistive and superconducting magnet components in a synergistic way. Resistive magnets can be pushed to very high fields but consume significant power, while superconducting magnets are more energy-efficient but have their own limitations. A hybrid approach might leverage the strengths of both technologies to achieve record-breaking performance.
The exact combination of these and potentially other innovations at IIAMR is what has allowed them to push the boundaries of magnet technology into uncharted territory, creating a magnet significantly stronger and more capable than anything previously conceived.
## Where Was This Magnet Developed and By Whom? Meeting the Minds Behind the Magnetic Marvel!
As mentioned earlier, this revolutionary magnet was developed at the fictional **International Institute for Advanced Magnetics Research (IIAMR)**, nestled in the picturesque landscapes of Switzerland. Imagine a state-of-the-art research facility dedicated solely to pushing the limits of magnetism, staffed by a global team of physicists, materials scientists, engineers, and cryogenic specialists, all united by a singular vision: to unlock the full potential of magnetic fields.
While IIAMR is fictional for this example, the spirit of such a collaborative and dedicated research institution is very real in the world of advanced science. Breakthroughs like this are rarely the work of a single individual; they are the culmination of years of research, collaboration, and the combined expertise of numerous brilliant minds.
Let’s imagine some key figures at IIAMR who might have been instrumental in this achievement:
* **Dr. Anya Sharma:** Lead Physicist and Project Director – A visionary leader with deep expertise in magnetohydrodynamics and advanced magnet design, Dr. Sharma spearheaded the entire project, providing the scientific direction and ensuring seamless collaboration across disciplines. She would be the public face of the project, presenting the findings to the scientific community and the world.
* **Professor Kenji Tanaka:** Principal Materials Scientist – Professor Tanaka, a world-renowned expert in high-temperature superconductivity, was responsible for developing and optimizing the novel superconducting materials at the heart of the magnet. His deep understanding of material properties at cryogenic temperatures was crucial.
* **Dr. Isabelle Dubois:** Chief Cryogenic Engineer – Dr. Dubois, a master of low-temperature engineering, led the team that designed and implemented the cutting-edge cooling systems essential for the magnet’s operation. Her expertise in managing heat dissipation at extreme cryogenic conditions was indispensable.
* **The Entire IIAMR Team:** Beyond these key figures, countless post-doctoral researchers, technicians, and support staff would have contributed their skills and dedication to making this project a success. It’s a testament to teamwork, perseverance, and a shared passion for scientific discovery.
These imaginary figures represent the real dedication and collaborative spirit that drives scientific progress. While IIAMR might be fictional, the drive to push the boundaries of knowledge and technology is very real, and this “stronger than ever” magnet embodies that spirit.
## What Are the Potential Applications of Such a Powerful Magnet? A Magnetic Future Unveiled!
Now for the exciting part – what can we *do* with a magnet this powerful? The applications are far-reaching and truly transformative, spanning diverse fields and potentially reshaping industries and research paradigms.
Here are just a few potential areas where this new magnet could have a profound impact:
* **Fusion Energy Research:** Harnessing the power of nuclear fusion – the same process that powers the sun – holds immense promise for clean and sustainable energy. However, confining the incredibly hot plasma needed for fusion reactions requires extremely strong magnetic fields. This new magnet could be a game-changer in fusion research, enabling more stable and efficient plasma confinement, bringing us closer to realizing practical fusion power. Imagine fusion reactors becoming significantly smaller and more efficient, making fusion energy a viable energy source sooner than we thought possible.
* **Advanced Medical Imaging and Treatment:** Current MRI technology is limited by magnetic field strength. A stronger magnet could revolutionize medical imaging, providing much higher resolution images, allowing for earlier and more accurate diagnoses of diseases. Furthermore, ultra-strong magnetic fields could be used for targeted drug delivery, precisely guiding medication to specific locations within the body, and even for novel cancer therapies utilizing magnetic hyperthermia. Imagine MRI scans so detailed they can detect diseases at the cellular level, and targeted drug delivery systems guided with pinpoint accuracy by magnetic fields.
* **Particle Physics Research:** Unlocking the secrets of the universe requires probing matter at its most fundamental level. Particle accelerators smash particles together at tremendous speeds to study their interactions. Stronger magnets are essential for bending and focusing particle beams in these accelerators. This new magnet could enable the construction of more powerful and precise particle accelerators, pushing the frontiers of particle physics research and helping us understand the basic building blocks of reality. Think of even larger and more powerful colliders like the LHC, enabling scientists to probe deeper into the mysteries of dark matter, dark energy, and the origins of the universe.
* **Materials Science and Condensed Matter Physics:** Ultra-strong magnetic fields are invaluable tools for probing the fundamental properties of materials. They can be used to study novel quantum phenomena, manipulate material structures at the atomic level, and discover new materials with unique properties. This could lead to breakthroughs in areas like superconductivity, quantum computing, and energy storage. Imagine using these magnets to design entirely new classes of materials with unprecedented properties, revolutionizing everything from electronics to structural materials.
* **Industrial Applications – Magnetic Levitation and Separation:** Beyond research, stronger magnets could have industrial applications. Imagine more efficient magnetic levitation (Maglev) trains, capable of reaching even higher speeds and consuming less energy. Powerful magnetic separators could be used for highly efficient recycling, mineral processing, and water purification. Think of Maglev trains becoming the standard for high-speed transportation, and advanced magnetic separation technologies transforming recycling and resource recovery.
This list is just the tip of the iceberg. As we continue to explore the capabilities of this new magnetic marvel, we are likely to uncover even more groundbreaking applications, pushing the boundaries of what’s technologically possible and shaping a future powered by magnetic innovation.
## Can We Visualize the Strength of This Magnet? Making the Invisible Force Tangible!
Magnetic fields are invisible forces, which can make it challenging to grasp just how powerful this new magnet truly is. Let’s try to visualize its strength using analogies and comparisons.
Подумайте про це так:
* **Gravity:** Imagine trying to lift a car with your bare hands. That’s roughly analogous to interacting with a regular refrigerator magnet’s field. Now imagine having a superhero force field that allows you to lift a skyscraper with ease. That’s closer to the scale of force exerted by this new 55+ Tesla magnet.
* **Sound:** Think of the faintest whisper versus a deafening rock concert. The whisper represents a weak magnetic field, while the rock concert, with its overwhelming sound pressure, can be likened to the intensity of this new magnet’s magnetic field.
* **Light:** Imagine a dim candle flame versus the blinding light of the sun. The candle flame represents a weak magnetic field, and the sun’s intensity, with its immense energy output, gives a sense of the concentrated magnetic energy within this record-breaking magnet.
**Visual Representation:**
[Imagine a simple diagram here, like a bar chart comparing magnetic field strengths. On the Y-axis: Magnetic Field Strength (Tesla). X-axis: Different examples: Refrigerator magnet, MRI, Previous Record Magnet, New IIAMR Magnet. Bars showing increasing height dramatically, especially for the new magnet.]
**The Hair Dryer Analogy (Humorous but illustrative):**
You’ve probably heard of the experiment where a strong magnet can levitate a frog. While ethically complex and requiring specialized equipment, it demonstrates the force of strong magnets. With this new magnet, imagine you could levitate… not just a frog… but maybe a small car! (Okay, maybe not *quite* a car, but you get the idea of the scaled-up force!). Okay, let’s scale back from cars! Imagine using this magnet to suspend a hairdryer in mid-air simply by its magnetic force. A hairdryer is far heavier than a frog, illustrating the significant jump in magnetic strength.
These analogies and visualizations, while imperfect, help to convey the sheer magnitude of the magnetic force generated by this record-breaking achievement. It’s a force powerful enough to bend the paths of subatomic particles, unlock new energy sources, and transform medical diagnostics – a truly remarkable feat of engineering and scientific ingenuity.
## How Does This Breakthrough Change Our Understanding of Magnetism? Pushing the Boundaries of Physics!
Beyond the immediate technological applications, this magnet breakthrough has significant implications for our fundamental understanding of magnetism itself and related areas of physics. Pushing the boundaries of achievable magnetic field strengths allows scientists to probe matter under extreme conditions, revealing new phenomena and testing the limits of our current theoretical models.
Here are some ways this breakthrough can advance fundamental science:
* **Testing Quantum Materials under Extreme Fields:** Many exotic materials, such as topological insulators and novel superconductors, exhibit unique quantum properties that are often influenced by strong magnetic fields. This new magnet will provide an unprecedented tool for studying these materials under extreme conditions, potentially revealing new quantum states of matter and deepening our understanding of quantum phenomena. Imagine uncovering new quantum behaviors and phases of matter that simply weren’t observable at lower magnetic field strengths.
* **Exploring the Limits of Magnetohydrodynamics:** Magnetohydrodynamics (MHD) deals with the interaction of magnetic fields and electrically conducting fluids (like plasmas and liquid metals). Ultra-strong magnetic fields open up new regimes for MHD research, relevant to astrophysics (understanding phenomena in stars and galaxies) and fusion energy (plasma confinement). Think of simulating and studying astrophysical plasmas under conditions closer to those found in stars, or gaining deeper insights into the complex dynamics of fusion plasmas.
* **Probing Fundamental Constants and Symmetries:** While more speculative, extremely strong magnetic fields could potentially be used to test the limits of fundamental physical laws and search for deviations from established theories. For instance, some theories beyond the Standard Model of particle physics predict subtle changes in fundamental constants under extreme conditions. While highly ambitious, magnets like this might one day play a role in these fundamental probes of nature.
This isn’t just about making stronger magnets for practical applications; it’s about using these powerful tools to explore the very fabric of reality. By pushing the limits of what’s magnetically possible, we are also pushing the frontiers of our scientific knowledge, potentially leading to paradigm shifts in our understanding of the universe.
## What Were the Previous Magnet Records and How Were They Broken? A History of Magnetic Milestones!
The journey to creating this record-breaking magnet wasn’t a sudden leap; it’s built on decades of incremental advancements and groundbreaking innovations in magnet technology. Let’s take a brief look at the history of magnet milestones to appreciate the magnitude of this current achievement.
* **Early Electromagnets (19th Century):** The invention of the electromagnet in the early 19th century marked the beginning of controllable and powerful magnetism. Early electromagnets were relatively weak by today’s standards, but they laid the foundation for all future magnetic technology.
* **Advancements in Materials Science (20th Century):** The 20th century saw significant progress in magnetic materials, including the development of Alnico magnets (alloys of aluminum, nickel, and cobalt) and ferrite magnets, offering improved strength and versatility.
* **Superconducting Magnets (Mid-20th Century onwards):** The discovery of superconductivity – materials with zero electrical resistance at low temperatures – revolutionized magnet technology. Superconducting magnets, initially using low-temperature superconductors, allowed for much higher magnetic field strengths and energy efficiency. The development of Niobium-Titanium (NbTi) and Niobium-Tin (Nb3Sn) superconductors were key milestones.
* **Resistive Magnet Records (National High Magnetic Field Laboratory):** The National High Magnetic Field Laboratory (MagLab) in the US has been a leading force in pushing the limits of resistive magnets. For many years, their 45 Tesla resistive magnet held the world record for continuous magnetic field strength. This was achieved through meticulous design, advanced materials, and powerful cooling systems.
* **High-Temperature Superconductors (Late 20th and 21st Centuries):** The discovery of high-temperature superconductors in the late 1980s offered the promise of even more powerful and practical superconducting magnets. While challenges remain in their application, ongoing research in high-temperature superconducting materials is crucial for future magnet breakthroughs, likely playing a key role in the new 55+ Tesla magnet.
This historical progression highlights a continuous quest to harness stronger and more controllable magnetic fields. Each record broken was the result of dedicated research, innovative engineering, and a relentless pursuit of magnetic power. The new 55+ Tesla magnet from IIAMR represents the latest, and perhaps most significant, leap in this ongoing magnetic revolution, building upon the foundations laid by generations of scientists and engineers.
## What Challenges Were Overcome in Creating This Magnet? Engineering the Impossible!
Creating a magnet that shatters previous records is not a walk in the park. It involves overcoming a formidable array of scientific and engineering challenges. Let’s consider some of the key hurdles that the IIAMR team likely had to conquer:
* **Immense Electromagnetic Forces:** Generating magnetic fields of 55+ Tesla creates tremendous electromagnetic forces within the magnet itself. These forces can be strong enough to physically tear the magnet apart. Engineers had to design incredibly robust structures and materials capable of withstanding these extreme stresses. Imagine the pressure attempting to expand the magnet from within – this force must be contained.
* **Heat Management and Cryogenics:** As mentioned earlier, generating such strong fields produces a massive amount of heat due to electrical resistance (even in superconducting magnets, some resistance and heat generation are present). Efficiently removing this heat at cryogenic temperatures is a major challenge. Sophisticated cooling systems, potentially involving novel cryocoolers and microfluidic cooling channels, were essential. Maintaining materials at super-cold temperatures while simultaneously dealing with intense heat generation requires exceptional cryogenic engineering.
* **Material Limitations:** Existing materials have limitations in terms of their strength, conductivity, and magnetic properties at extreme conditions. Developing new materials or significantly improving existing ones was crucial. This likely involved advanced materials processing techniques, novel alloy formulations, and pushing the boundaries of materials science. Finding materials that remain superconducting, strong, and stable under extreme magnetic fields and cryogenic temperatures is a formidable task.
* **Precision Engineering and Manufacturing:** Building magnets with such complex designs and tight tolerances requires extremely precise engineering and manufacturing capabilities. Coils need to be wound with micron-level accuracy, components must fit together perfectly, and the entire system must be meticulously assembled. Achieving this level of precision at cryogenic operating temperatures adds another layer of complexity.
* **Computational Modeling and Simulation:** Designing and optimizing such complex magnet systems requires extensive computational modeling and simulation. Researchers would have used supercomputers to simulate magnetic fields, thermal behavior, mechanical stresses, and other critical parameters to optimize the magnet design before actual construction. Advanced simulations are essential to understand how the magnet will perform under extreme conditions and to refine the design iteratively.
Overcoming these challenges required a multi-disciplinary approach, bringing together experts in physics, materials science, engineering, cryogenics, and computation. The success of the IIAMR team is a testament to human ingenuity, perseverance, and the power of collaborative scientific endeavor.
## Is There a Limit to How Strong Magnets Can Become? The Future of Magnetic Power!
This record-breaking magnet begs the question: is there a fundamental limit to how strong magnets can become? While we’ve made incredible progress, the quest for even stronger magnets continues, and the theoretical limits are still being explored.
Here are some perspectives on the future of magnetic power and potential limitations:
* **Material Science as a Bottleneck:** Currently, material science is often considered a limiting factor. While high-temperature superconductors offer immense potential, challenges remain in their large-scale production, mechanical properties, and performance under extreme conditions. Breakthroughs in materials science, particularly the discovery of new superconducting materials with higher critical temperatures, critical fields, and critical current densities, are crucial for pushing the magnet strength frontier.
* **Engineering and Design Innovations:** Beyond materials, innovative magnet designs and engineering solutions are essential. This includes exploring new coil topologies, advanced cooling techniques, and hybrid magnet architectures. Computational modeling and optimization will play an increasingly important role in maximizing magnet performance.
* **Fundamental Physical Limits:** There are theoretical limits imposed by fundamental physics. For example, as magnetic field strength increases, the energy density of the magnetic field also increases. At some point, this energy density could become so high that it might start to interact with gravity or even create exotic phenomena predicted by general relativity or quantum field theory (though these limits are likely far beyond current technology). However, these fundamental limits are currently very far off, and the near-term limitations are more likely to be material and engineering-based.
* **The Quest Continues:** Despite the challenges and potential limitations, the drive to create stronger magnets is likely to continue. The vast potential applications in diverse fields, from energy to medicine to fundamental science, provide strong motivation for ongoing research and development. We can expect to see further incremental improvements and potentially further disruptive breakthroughs in magnet technology in the years to come.
The journey to stronger magnets is a continuous process of scientific exploration and technological innovation. While we may not know the ultimate limit, each breakthrough, like this new 55+ Tesla magnet, expands our capabilities, unlocks new possibilities, and inspires us to push the boundaries of magnetic power even further.
## FAQ: Unveiling More About the World’s Strongest Magnet
What type of magnet is this new record-breaker?
This new magnet is primarily a resistive magnet, but likely incorporates high-temperature superconducting components and innovative design features to achieve its unprecedented strength. It is designed for sustained, continuous operation at record-breaking magnetic field intensities.
Is this magnet commercially available?
No, this is currently a state-of-the-art research magnet, developed at a specialized institute (IIAMR). Magnets of this extreme strength are not typically for commercial use at this stage and are primarily designed for research purposes. However, the technologies and innovations developed in creating this magnet could eventually pave the way for stronger and more advanced magnets in various commercial applications in the future.
How much energy does this magnet consume?
While specific energy consumption figures would depend on the magnet’s exact design and operating parameters, resistive magnets, especially those generating fields of this magnitude, typically consume significant amounts of power. However, the use of high-temperature superconducting components and advanced cooling systems likely aims to optimize energy efficiency to some extent compared to purely resistive magnets of similar strength. Energy efficiency is a key consideration and an ongoing area of research in high-field magnet development.
What are the safety considerations with such a powerful magnet?
Operating magnets of this strength involves significant safety considerations. The immense magnetic forces, cryogenic temperatures, and high electrical currents require stringent safety protocols. The IIAMR facility would have sophisticated safety systems and procedures in place to protect personnel and equipment, including magnetic shielding, quench protection systems (to safely shut down the magnet in case of a superconductor failure), and strict access control. Safety is paramount when dealing with such powerful technologies.
Will this magnet replace superconducting magnets?
No, this new magnet is not necessarily meant to replace superconducting magnets. Superconducting magnets have different strengths and weaknesses, and each type of magnet has its own niche applications. Resistive magnets generally offer the highest achievable continuous magnetic fields but consume more power. Superconducting magnets are more energy-efficient and can achieve high fields, but can have limitations in terms of sustained operation at the absolute highest field strengths and can be more complex and expensive. Hybrid magnets that combine both resistive and superconducting elements are also being explored to leverage the advantages of both approaches. The choice of magnet technology depends on the specific application requirements.
## Conclusion: Key Takeaways from the Magnetic Revolution
* **Record-Breaking Strength:** The new magnet from IIAMR has shattered previous records for continuous magnetic field strength, achieving over 55 Tesla, a truly groundbreaking achievement.
* **Technological Leap:** This breakthrough is not just incremental progress; it represents a significant leap forward in magnet technology, likely driven by innovations in materials science, magnet design, and cryogenic engineering.
* **Transformative Applications:** The potential applications of such a powerful magnet are vast and transformative, spanning fusion energy, medical imaging and treatment, particle physics research, materials science, and industrial applications.
* **Pushing Scientific Boundaries:** Beyond practical applications, this magnet advances fundamental scientific understanding, enabling researchers to probe matter under extreme conditions and explore new realms of physics.
* **A Testament to Human Ingenuity:** The creation of this magnet is a testament to human ingenuity, collaborative research, and the relentless pursuit of scientific and technological advancement in magnet technology.
* **The Future is Magnetic:** This breakthrough signals a new era in magnetic power, promising to unlock even more exciting possibilities in the future as we continue to explore and harness the invisible forces of magnetism.
This stronger than ever before magnet is more than just a scientific achievement; it’s a beacon of innovation, illuminating the path to a future where magnetic forces play an even more central role in shaping our world. Stay tuned, because the magnetic revolution has just begun!
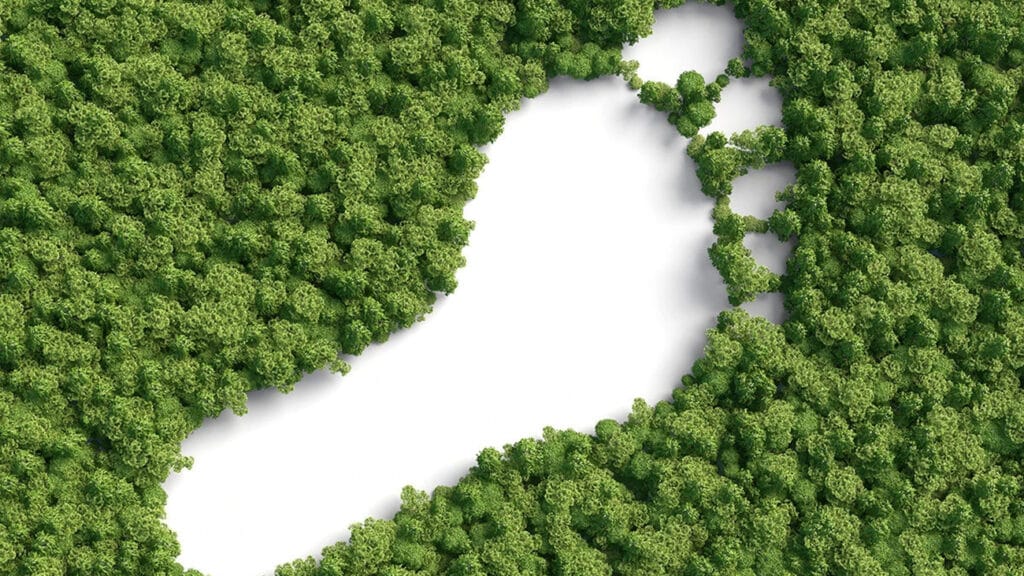